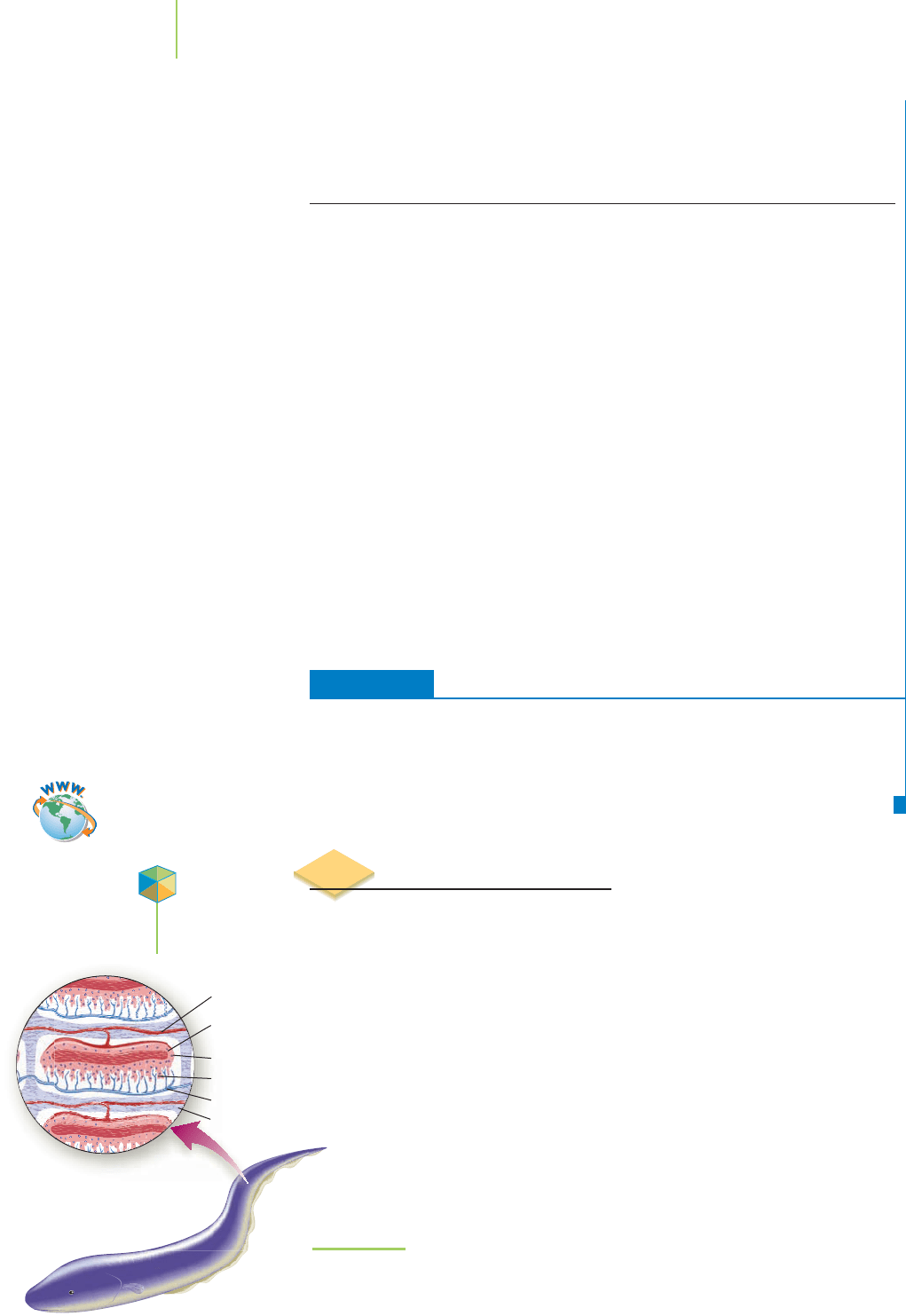
Connective tissue
Nerve
Papillae
Nucleus
Blood supply
Electrically conductive
tissue
Application
C
HEMICAL
ENCOUNTERS:
The Electric Eel
being oxidized. We can use the potentials of the half-reactions to calculate the
cell voltage.
Au(s) n Au
3+
(aq) + 3e
−
E° =−1.50 V
3e
−
+ 3H
+
(aq) + HNO
3
(aq) n NO(g) + 2H
2
O(l) E° =+0.96 V
3H
+
(aq) +HNO
3
(aq) +Au(s) n NO(g) +Au
3+
(aq) +2H
2
O(l) E°
cell
=−0.54 V
The negative value for the cell voltage indicates that the reaction of nitric acid and
gold is not spontaneous. Nitric acid doesn’t dissolve gold.
Further Insights
You’ve probably noticed that you don’t have to balance a redox reaction in order to
determine the standard voltage of the reaction. This is because the voltage of a reac-
tion is not dependent on the stoichiometry of the equation. Simply adding the half-
reaction potentials (as long as one is an oxidation and the other a reduction) yields
the voltage of the resulting reaction. However, it is good practice to balance the
equation so that we can tell how many electrons are exchanged. We will need this in-
formation if our system is at nonstandard conditions, which is most of the time.
A process for dissolving gold was known during the early days of the alchemists.
Because metals like gold and platinum wouldn’t react with strong acids, such as ni-
tric acid, they were known as the royal metals. However, there existed a solution that
would dissolve gold and platinum. These metals were soluble in a mixture of one
part nitric acid and three parts hydrochloric acid known as aqua regia, or royal
water. Aqua regia is used commercially as a first step in separating platinum from
gold that is combined with other metals in their ore mixtures. Both metals are ini-
tially dissolved and then selectively precipitated from solution.
PRACTICE 19.6
Determine the cell voltage at standard conditions for the following redox reactions:
CH
4
(g) + H
2
O(g) n CO(g) + H
2
(g)
Ag(s) + H
2
O
2
(aq) n H
2
O(l) +Ag
+
(aq)
See Problems 27 and 28.
19.4 Electrochemical Cells
It may be “shocking” to learn, but it’s true: The electric eel is a formidable oppo-
nent. When startled, stepped on, or hunting for food, the eel can deliver up to
1 ampere (1 coulomb of charge per second) at 600 V, enough electrical energy
to stun or even kill large animals. (To be accurate, the electric eel isn’t
an eel. It lives in fresh water and is really a fish.) How does the electric
eel generate the electricity used in hunting?
The powerful shock that the eel can deliver is produced by 5000 to
10,000 specialized cells, called electroplates, in its tail (see Figure 19.10).
Using biochemical processes, the eel charges up the electroplates in
much the same manner as muscle and nerve cells are charged. Then,
when a nerve impulse is sent to the tail, the electroplates discharge their
stored potential. Can you think of an electrical storage device similar to
the electric eel’s that we use every day? The electrochemical cell seems to
fit the definition. We’ll explore the electrochemical cell in this section
844 Chapter 19 Electrochemistry
FIGURE 19.10
Electroplates in the tail of the electric eel develop a potential charge that
can be delivered to shock its prey.
(Drawing by Rick Simonson.)
Video Lesson: Electrochemical
Cells