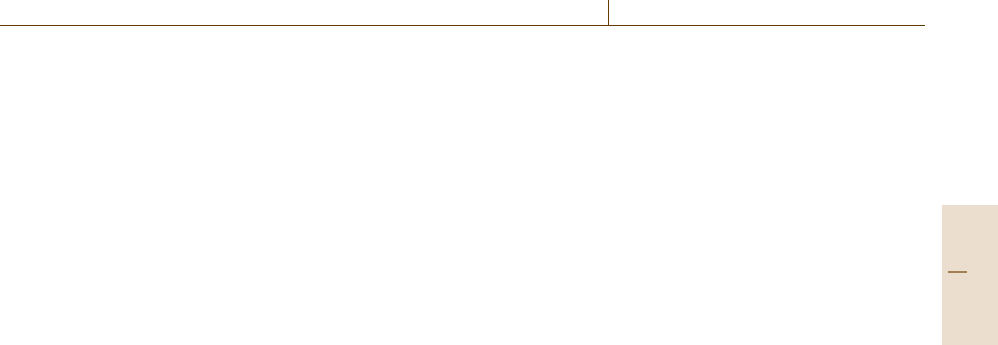
Nanoscopic Architecture and Microstructure 5.3 Lattice Defects and Impurities Analysis 239
to unoccupied upper levels. The core absorption spec-
trum has a characteristic fine structure extending over
hundreds of eV above the absorption edge. The undu-
lating structures within ≈ 50 eV of the edge is referred
to as x-ray absorption near-edge structures (XANES) or
near-edge x-ray absorption fine structures (NEXAFS),
whereas the fine structures beyond ≈ 50 eV are called
the extended x-ray absorption fine structures (EXAFS).
The EXAFS is brought about by interference of the
excited electron wave primarily activated at a core
with the secondary waves scattered by the surround-
ing atoms (Fig. 5.46). Therefore, the EXAFS contains
information on the local atomic arrangement, neigh-
bor distances and the coordination numbers, around the
atoms of a specific element responsible for the core
excitation absorption. Figure 5.47a shows an experi-
mental x-ray absorption spectrum near the zinc K-edge
of an amorphous Mg
70
Zn
30
alloy, and Fig. 5.47bthe
oscillatory part of the EXAFS obtained by subtracting
the large monotonic background in (a). Assuming ap-
propriate phase shifts on electron scattering, we obtain
Fig. 5.47c, a Fourier transform of a function modified
from (b), from which we deduce Fig. 5.47d, the par-
tial distribution function around Zn atoms. The area of
deconvoluted peaks in (d) gives the coordination num-
ber for the respective atom pair. The element-selectivity
of the EXAFS method provides a unique tool that en-
ables one to investigate the local order for different
atomic species in alloys, regardless of the long-range
order or the crystallinity and amorphousness of the
sample.
X-Ray Photoemission Spectroscopy (XPS)
Though x-ray photoemission spectroscopy (XPS)is
surface sensitive, it may be used for studies of very
short-range order around atoms. The energy of the pho-
toelectrons measured relative to the Fermi level of the
sample directly reflects the energy of the core elec-
trons. The core level is slightly affected by how much
electronic charge is distributed on the nucleus in the
solid (chemical shift): the more negatively charged, the
higher the core level and hence the smaller the pho-
toelectron energy. The spectral peak position and its
intensity in XPS thus indicate the character of chemical
bonding or the degree of chemisorption to the selec-
tively probed atoms.
Raman Scattering
Since the principle of Raman scattering has been
described already (Sect. 5.1.2), we only mention an ap-
plication of Raman scattering to studies of structural
order in crystalline and amorphous solids. From the
energy of Raman-active optical phonons, the material
phases present in the sample can be determined. The
selection rule in infrared absorption based on the trans-
lational symmetry of the crystal allows only optical
modes near k ≈0 in the Brillouin zone. Similarly the
translational symmetry restricts the Raman scattering to
limited modes. The presence of defects or disorder in
crystals breaks the translational symmetry of the lattice,
so that translationally forbidden Raman modes become
active and detectable as an increase of the extended
lattice phonon signal. Raman scattering is one of the
standard methods for structural assessment of graphitic
or amorphous carbon [5.36]. This is owing to the fact
that the visible spectral region, the most widely used
for Raman scattering experiments, is electronically res-
onant with graphitic crystals, enhancing the scattering
signals. Two broad spectral bands, called G and D, re-
spectively corresponding to crystalline and disordered
graphitic phases are well separated for quantitative anal-
ysis of disorder.
5.3 Lattice Defects and Impurities Analysis
This section deals with methods for the study of atom-
istic structural defects and impurities in regular lattices.
Section 5.3.1 covers point defects including vacancies,
interstitial atoms, defect complexes, defect clusters, and
impurities. Section 5.3.2 deals with extended defects in-
cluding dislocations, stacking faults, grain boundaries,
and phase boundaries.
The sensitivity (or field of view) of the methods de-
scribed here is not necessarily high (wide) enough for
detecting a low density of defects, so in many cases the
defects must be intentionally introduced into the sample
by some means. For intrinsic point defects such as va-
cancies and self-interstitials, the most common method
is to irradiate the sample with high energy particles such
as MeV electrons and fast neutrons. For dislocations, the
sample may have to be deformed plastically. However,
one should always pay careful attention to the possibil-
ity that the primary defects may react to form complexes
with themselves or impurities and the plastically de-
formed samples may contain point defects as well.
Part B 5.3