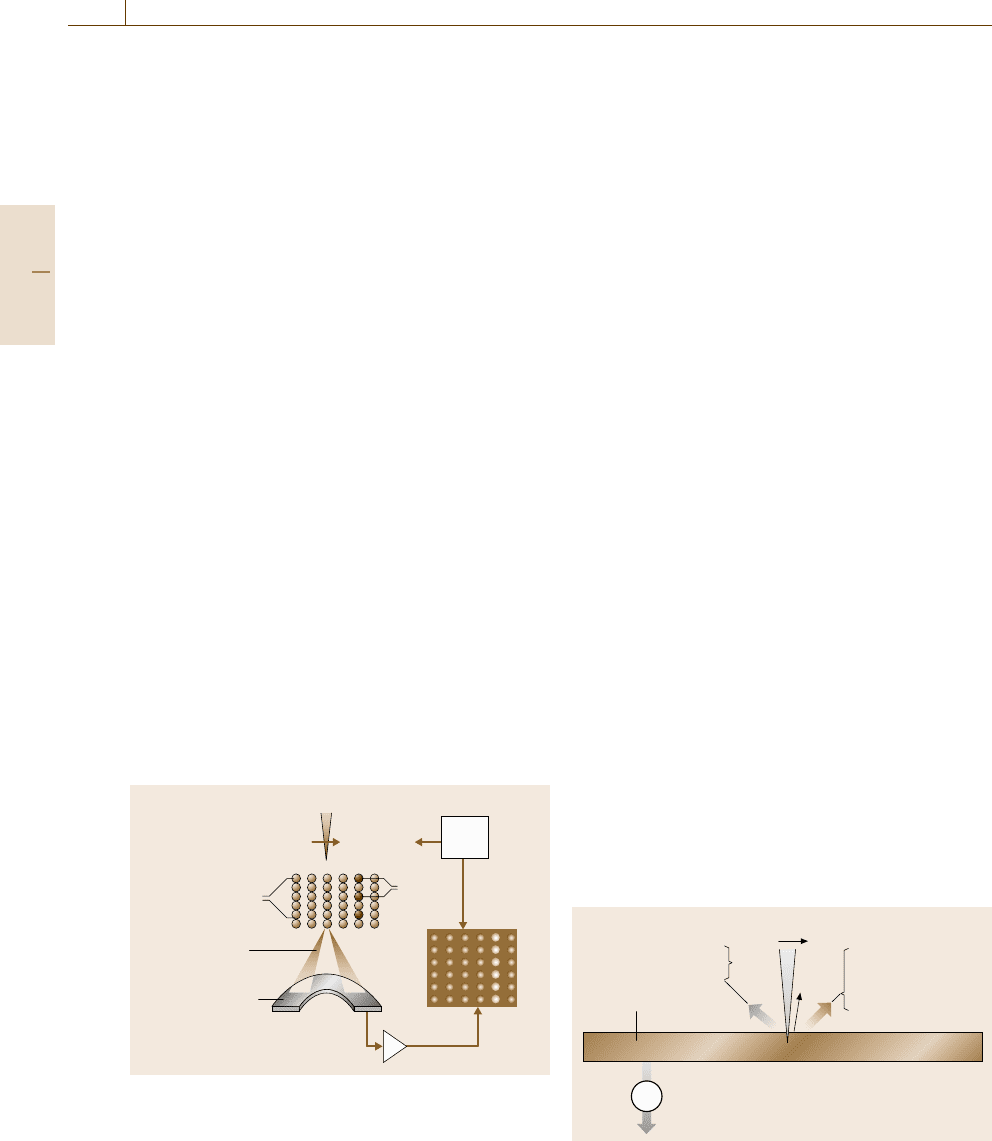
222 Part B Chemical and Microstructural Analysis
In bright-field (BF) STEM, the signal collected is
the directly transmitted electrons that are not diffracted
by the crystallographic sample. Similarly, dark-field
(DF) STEM images can be obtained by collecting only
diffracted electrons. Although the imaging principle of
STEM is different from that of TEM, the two micro-
scopic techniques could be viewed as equivalent if one
regards the electron detector in STEM as the objective
aperture in TEM, both of which are (not physically as
for the detector in STEM) placed at the back focal plane
of the objective lens.
High-angle annular dark-field STEM (HAADF-
STEM) allows one to observe STEM images with
higher resolution and compositional information. As
shown in Fig. 5.19, HAADF-STEM images are formed
by collecting high-angle scattered electrons with an an-
nular dark-field detector placed at the back focal plane
of the objective lens. The image formation mecha-
nism is based on the fact that a fraction of electrons
incident on a crystalline sample along a channeling
direction are diffusely scattered by thermal vibrations
of atoms at relatively high angles ( 70 mrad) when
the beam scans over the atomic columns. Therefore, in
HAADF-STM images, the positions of atoms are ob-
served as bright contrasts depending strongly on the
average atomic number of the atoms in the column.
Owing to this feature, HAADF-STM, otherwise called
Z-contrast imaging, is a powerful technique for compo-
sition analysis especially for elements of large atomic
numbers. The spatial resolution in HAADF-STEM is
limited by the size of the focused probe beam as in or-
Focused electron beam
Convergent angle
≈ 10 mrad
Probe size & 0.2 nm
Beam scan
Scan
circuit
Raster scan
2-D
Map
Small Z
Large Z
Scattering angle
& 70 mrad
Annular detector
Collected current
Fig. 5.19 Setup of high-angle annular dark-field scanning
transmission electron microscopy (HAADF-STEM). Elec-
trons diffusely scattered by thermal vibrations of atoms
with relatively high angles are collected by an annular de-
tector to form a scan image
dinary STEM. Under good conditions, an atomic-level
spatial resolution can be achieved, although such sam-
ples must be thin enough (usually thinner than several
tens of nanometers) for beam broadening to be avoided.
The advantage of HAADF-STM over HRTEM is the in-
sensitivity to focusing conditions and sample thickness
variations, both of which very substantially affect the
phase sensitive contrast of HRTEM images.
Scanning Electron Microscopy (SEM). The representa-
tive microscope of the scanning type is the scanning
electron microscope (SEM)[5.17], in which the scan-
ning probe is a finely focused electron beam. Fig-
ure 5.20 shows various forms of signals generated by
incidence of electrons onto solid samples. Some of the
incident electrons lose most of their initial energy and
are emitted from the surface with a low energy (≈afew
tens of eV). These electrons are called secondary elec-
trons, which can escape only from very shallow depths
of the solid due to their large absorption cross section
(Fig. 5.12). Without losing much energy, other electrons
are either reflected backward as back-scattered elec-
trons or absorbed into the solid as a drain current. On
the loss of electron energy, x-ray fluorescence or Auger
electron emission occur, which are very useful for elem-
ent analysis. In semiconductor samples, the electron
energy may be converted to a luminescence (cathodo-
luminescence, CL) or an electron-beam-induced current
(EBIC) when an electric field separating electron–hole
pairs is built up in the surface layer. Depending on the
detected signal, SEM has several operation modes. The
most common mode is secondary electron SEM (SEM-
SE), in which the difference in the escape probability of
secondary electrons depending on the direction relative
to the surface normal produces surface roughness con-
trast observed from an oblique angle when the electron
detector is placed as shown in Fig. 5.21.
Finely focused beam scan
X-ray fluorescence
Cathodoluminescence
Secondary electrons
Backward scattered
electrons
Auger electrons
Electron beam induced current
A
Sample
Fig. 5.20 Signals generated in scanning electron micro-
scopy
Part B 5.1