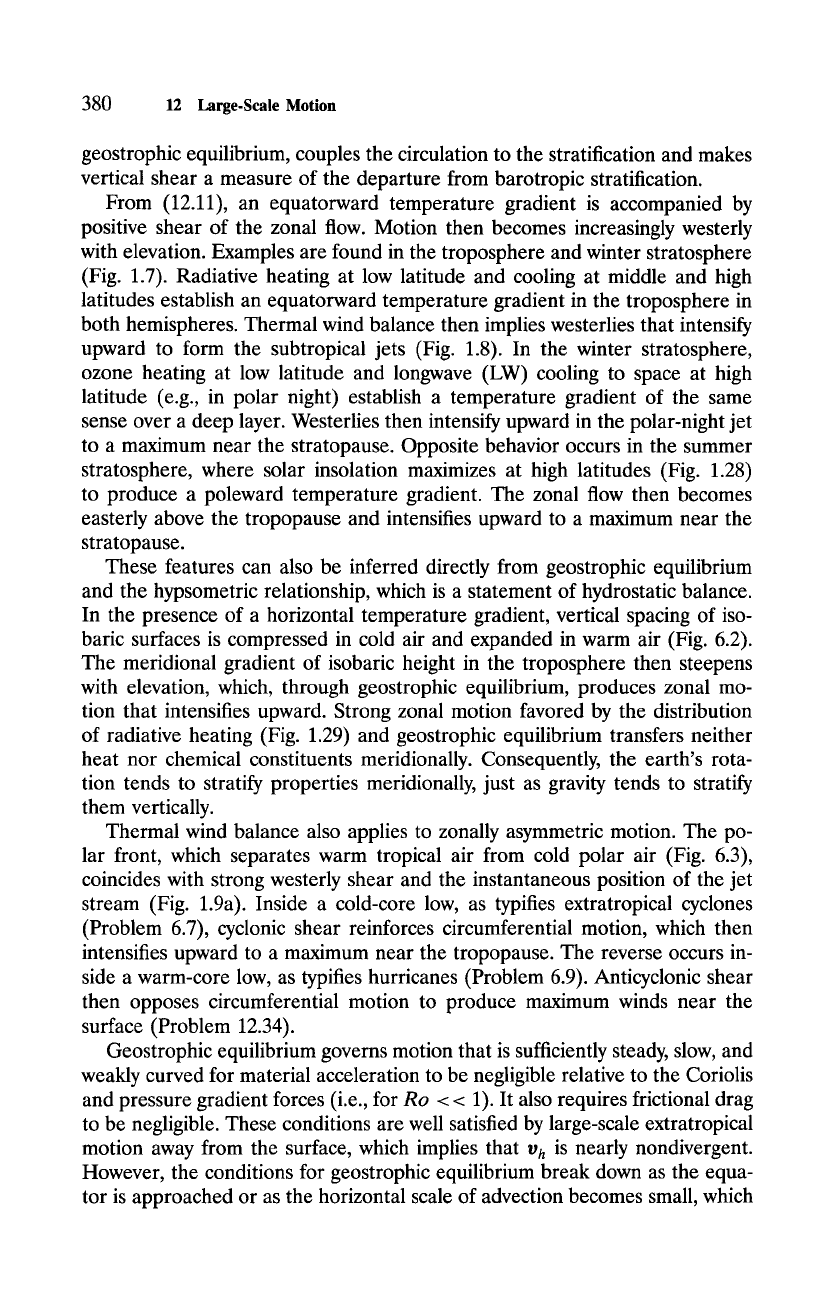
380
12 Large-Scale
Motion
geostrophic equilibrium, couples the circulation to the stratification and makes
vertical shear a measure of the departure from barotropic stratification.
From (12.11), an equatorward temperature gradient is accompanied by
positive shear of the zonal flow. Motion then becomes increasingly westerly
with elevation. Examples are found in the troposphere and winter stratosphere
(Fig. 1.7). Radiative heating at low latitude and cooling at middle and high
latitudes establish an equatorward temperature gradient in the troposphere in
both hemispheres. Thermal wind balance then implies westerlies that intensify
upward to form the subtropical jets (Fig. 1.8). In the winter stratosphere,
ozone heating at low latitude and longwave (LW) cooling to space at high
latitude (e.g., in polar night) establish a temperature gradient of the same
sense over a deep layer. Westerlies then intensify upward in the polar-night jet
to a maximum near the stratopause. Opposite behavior occurs in the summer
stratosphere, where solar insolation maximizes at high latitudes (Fig. 1.28)
to produce a poleward temperature gradient. The zonal flow then becomes
easterly above the tropopause and intensifies upward to a maximum near the
stratopause.
These features can also be inferred directly from geostrophic equilibrium
and the hypsometric relationship, which is a statement of hydrostatic balance.
In the presence of a horizontal temperature gradient, vertical spacing of iso-
baric surfaces is compressed in cold air and expanded in warm air (Fig. 6.2).
The meridional gradient of isobaric height in the troposphere then steepens
with elevation, which, through geostrophic equilibrium, produces zonal mo-
tion that intensifies upward. Strong zonal motion favored by the distribution
of radiative heating (Fig. 1.29) and geostrophic equilibrium transfers neither
heat nor chemical constituents meridionaUy. Consequently, the earth's rota-
tion tends to stratify properties meridionally, just as gravity tends to stratify
them vertically.
Thermal wind balance also applies to zonally asymmetric motion. The po-
lar front, which separates warm tropical air from cold polar air (Fig. 6.3),
coincides with strong westerly shear and the instantaneous position of the jet
stream (Fig. 1.9a). Inside a cold-core low, as typifies extratropical cyclones
(Problem 6.7), cyclonic shear reinforces circumferential motion, which then
intensifies upward to a maximum near the tropopause. The reverse occurs in-
side a warm-core low, as typifies hurricanes (Problem 6.9). Anticyclonic shear
then opposes circumferential motion to produce maximum winds near the
surface (Problem 12.34).
Geostrophic equilibrium governs motion that is sufficiently steady, slow, and
weakly curved for material acceleration to be negligible relative to the Coriolis
and pressure gradient forces (i.e., for
Ro
< < 1). It also requires frictional drag
to be negligible. These conditions are well satisfied by large-scale extratropical
motion away from the surface, which implies that
v h
is nearly nondivergent.
However, the conditions for geostrophic equilibrium break down as the equa-
tor is approached or as the horizontal scale of advection becomes small, which