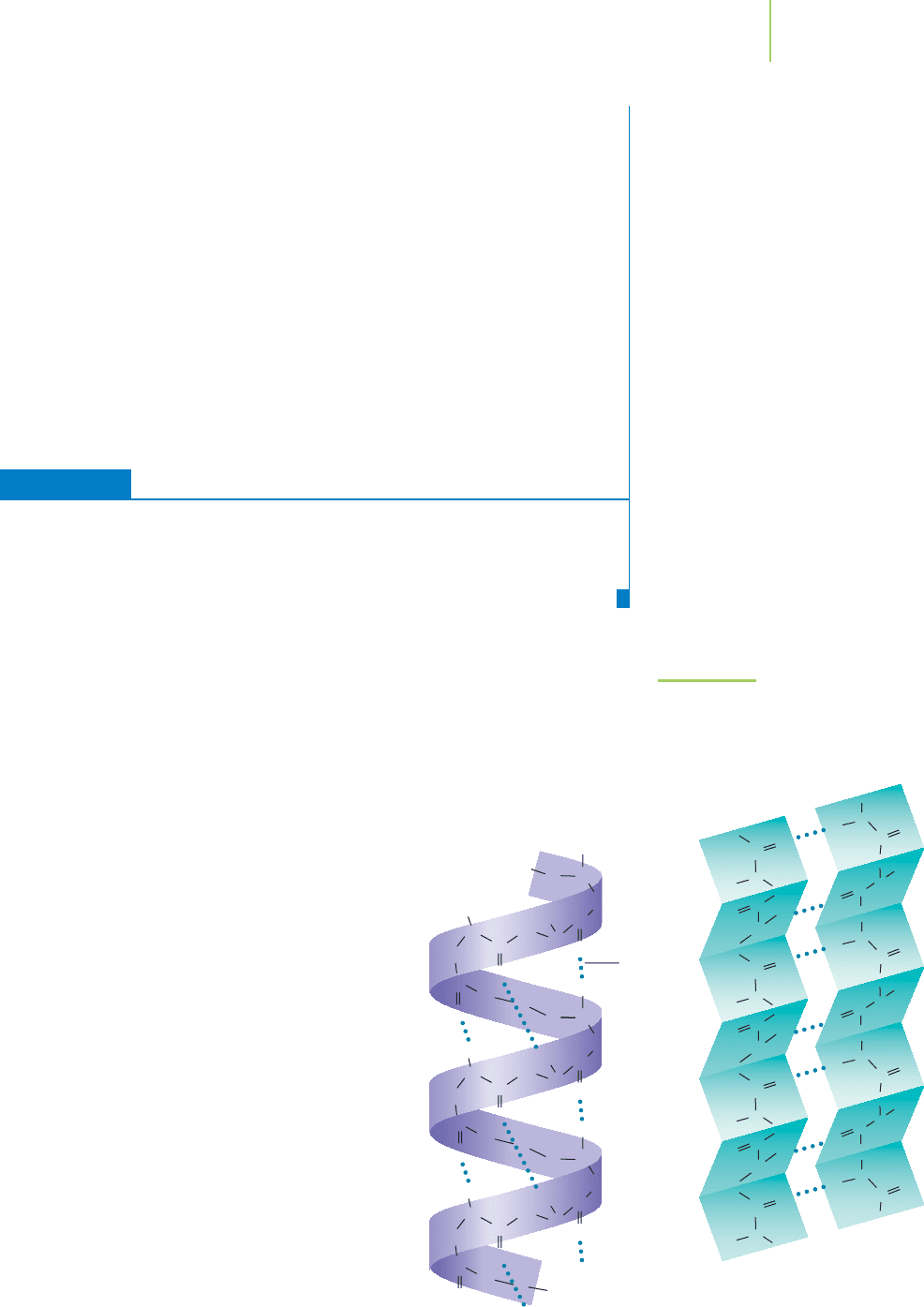
Solution
There are 64 possible codons—different ways to arrange the four bases into sets of
three. There are only about 20 amino acids found in proteins, so there must be more
codons available than amino acids. Examining the genetic code reveals that each
amino acid can be encoded by several alternative codons. It also reveals that three
codons act as “stop” signals, indicating the point at which the synthesis of a protein
chain should end.
Further Insights
Damage to DNA can result in changes to the genetic code. For example, a specific
base within a codon could be changed as a result of the damage. Some of these
changes have no effect on the protein encoded by the DNA, because they change a
codon into one of the other codons specifying the same amino acid. Other such
changes cause a different amino acid to appear in the encoded protein. This can
cause problems with the resulting protein that is made using the mutant codon.
PRACTICE 22.3
What is the primary structure of a protein made using the following mRNA
sequence?
AUGUGGCCAAAAUUGGACAUGUUCGACUAG
See Problems 15 and 16.
The human genome contains between about 20,000 and 30,000 genes. These
genes are able to encode an even greater number of proteins, because the RNAs
that are originally made from the genes can be edited to make many different
proteins in a kind of enzymic “cut-and-paste” process. The way in which genes
encode proteins is an astonishing demonstration of the power of chemistry to
sustain the complex processes that underpin all life. To understand more fully
just how powerfully genes influence the chemistry of life, we need to look at the
proteins encoded by our genes and investigate the things these proteins can do.
Protein Folding
As soon as a protein chain begins to be formed, it
starts to fold into a specific three-dimensional con-
formation. The folding process is governed princi-
pally by noncovalent interactions among the amino
acids themselves, and also among the amino acids
and the water molecules surrounding the protein.
Localized regions within a polypeptide chain that
fold in a particular way are examples of a protein’s
secondary structure. The most significant secondary
structures are the alpha helix ( helix) and the beta
pleated sheet ( sheet), shown in Figure 22.18. The
helix forms as a consequence of hydrogen bonds
between the NOH and CPO groups of the
polypeptide chain, holding the chain in the form of
a helix. The sheet is also held together by hydro-
gen bonds between NOH and CPO groups, but
with the hydrogen bonding occurring between
neighboring portions of a polypeptide chain.
Regions of specific secondary structure are linked
by turns in the polypeptide chain, and by less ordered
structures, to form the overall three-dimensional
22.3 How Genes Code for Proteins 945
FIGURE 22.18
Two common types of secondary struc-
tures: the alpha helix and the beta sheet.
These structures are held together by hy-
drogen bonding (shown as dotted lines).
C
C
N
N
N
N
H
H
H
H
O
O
O
C
C
C
C
O
C
C
C
O
C
C
N
N
N
N
H
Hydrogen
bonds
Alpha helix
H
H
H
O
O
O
C
C
C
C
O
C
C
C
C
N
N
N
N
H
H
H
H
O
O
O
C
C
C
C
C
N
N
N
O
O
O
H
H
H
H
N
C
C
C
C
C
C
O
C
C
Beta sheet
N
N
N
O
O
O
H
H
H
H
N
C
C
C
C
C
C
O
C
C
N
N
N
O
O
O
H
H
H
H
N
C
C
C
C
C
C
O
C
C
N
N
O
O
H
H
C
C
C
C
C
C