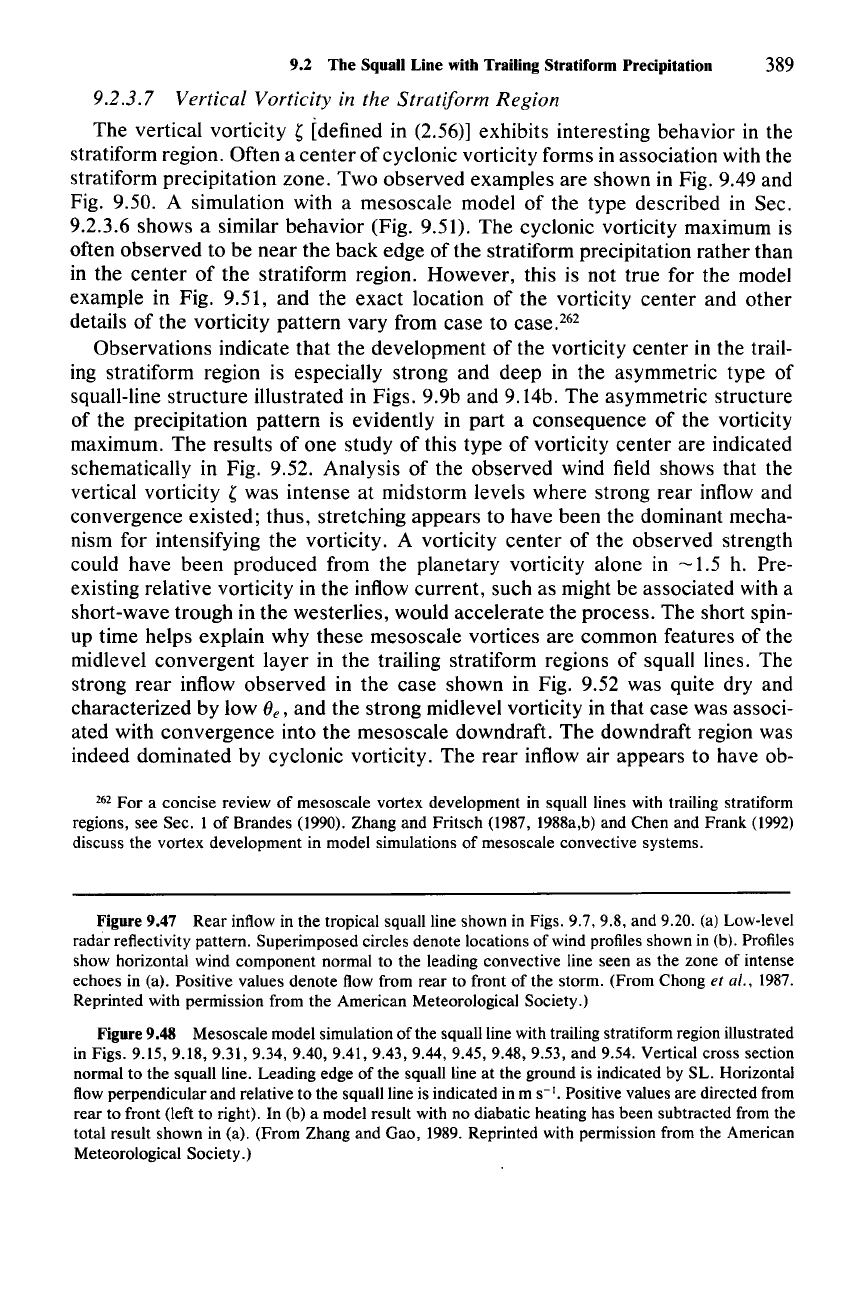
9.2 The Squall Line with Trailing Stratiform Precipitation 389
9.2.3.7 Vertical Vorticity in the Stratiform Region
The vertical vorticity
~
[defined in (2.56)] exhibits interesting behavior in the
stratiform region. Often a
center
of cyclonic vorticity forms in association with the
stratiform precipitation zone. Two observed examples are shown in Fig. 9.49 and
Fig. 9.50. A simulation with a mesoscale model of the type described in Sec.
9.2.3.6 shows a similar behavior (Fig. 9.51). The cyclonic vorticity maximum is
often observed to be
near
the back edge of the stratiform precipitation rather than
in the center
of
the stratiform region. However, this is not true for the model
example in Fig. 9.51, and the exact location of the vorticity center and other
details of the vorticity pattern vary from case to case.
262
Observations indicate that the development of the vorticity center in the trail-
ing stratiform region is especially strong and deep in the asymmetric type of
squall-line structure illustrated in Figs. 9.9b and 9.14b. The asymmetric structure
of the precipitation pattern is evidently in part a consequence of the vorticity
maximum. The results of one study of this type of vorticity center are indicated
schematically in Fig. 9.52. Analysis of the observed wind field shows that the
vertical vorticity
~
was intense at midstorm levels where strong rear inflow and
convergence existed; thus, stretching appears to have been the dominant mecha-
nism for intensifying the vorticity. A vorticity center of the observed strength
could have been produced from the planetary vorticity alone in
-1.5
h. Pre-
existing relative vorticity in the inflow current, such as might be associated with a
short-wave trough in the westerlies, would accelerate the process. The short spin-
up time helps explain why these mesoscale vortices are common features of the
midlevel convergent layer in the trailing stratiform regions of squall lines. The
strong
rear
inflow observed in the case shown in Fig. 9.52 was quite dry and
characterized by low
(Je, and the strong midlevel vorticity in that case was associ-
ated with convergence into the mesoscale downdraft. The downdraft region was
indeed dominated by cyclonic vorticity. The rear inflow air appears to have ob-
262 For a concise review of mesoscale vortex development in squall lines with trailing stratiform
regions, see Sec. I of Brandes (1990). Zhang and Fritsch (1987, 1988a,b) and Chen and Frank
(1992)
discuss the vortex development in model simulations of mesoscale convective systems.
Figure 9.47 Rear inflow in the tropical squall line shown in Figs. 9.7, 9.8, and 9.20. (a) Low-level
radar reflectivity pattern. Superimposed circles denote locations of wind profiles shown in (b). Profiles
show horizontal wind component normal to the leading convective line seen as the zone of intense
echoes in (a). Positive values denote flow from rear to front of the storm. (From Chong et al.,
1987.
Reprinted with permission from the American Meteorological Society.)
Figure 9.48 Mesoscale model simulation of the squall line with trailing stratiform region illustrated
in Figs. 9.15, 9.18, 9.31, 9.34, 9.40, 9.41, 9.43, 9.44, 9.45, 9.48, 9.53, and 9.54. Vertical cross section
normal to the squall line. Leading edge of the squall line at the ground is indicated by SL. Horizontal
flow perpendicular and relative to the squall line is indicated in m
S-I.
Positive values are directed from
rear to front (left to right). In (b) a model result with no diabatic heating has been subtracted from the
total result shown in (a). (From Zhang and Gao, 1989. Reprinted with permission from the American
Meteorological Society.)