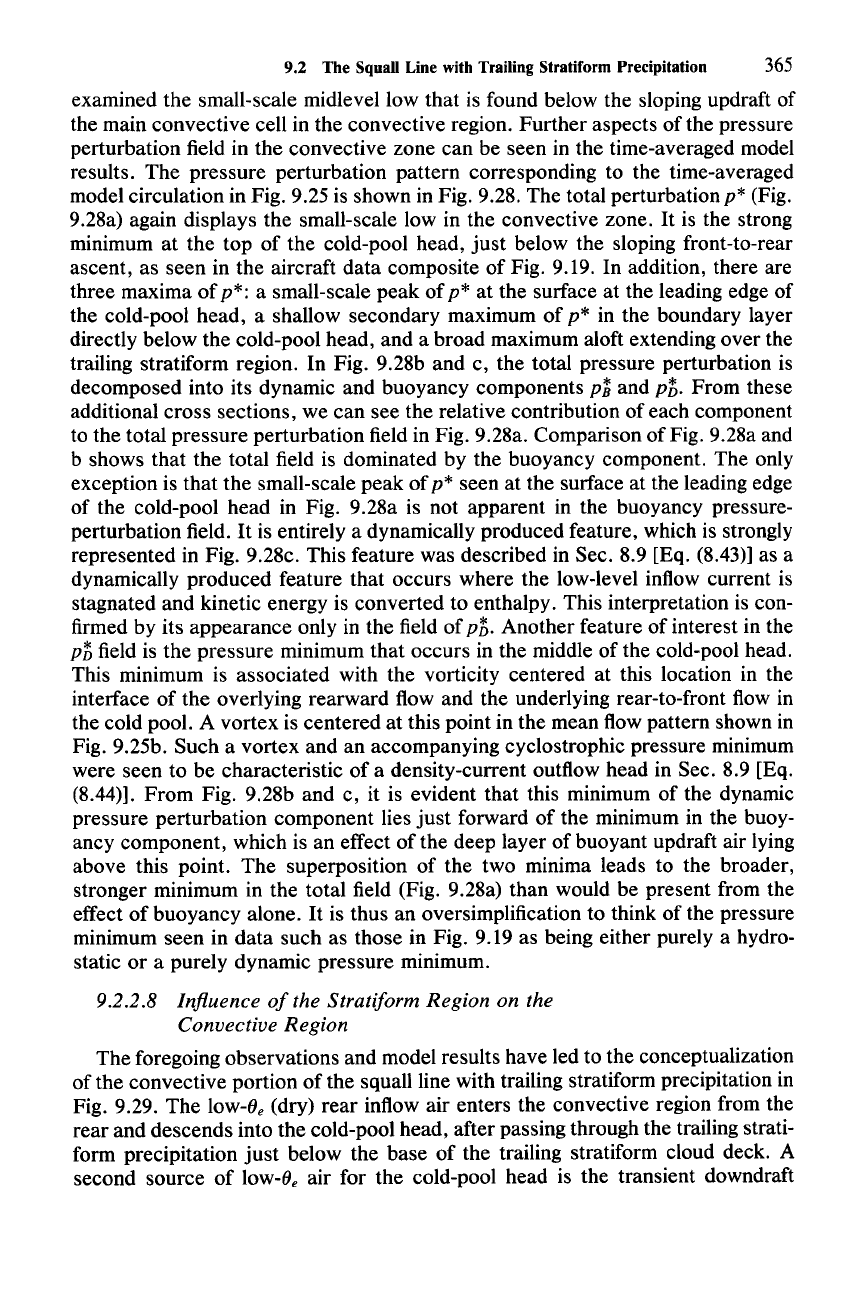
9.2 The Squall Line with Trailing Stratiform Precipitation
365
examined the small-scale midlevel low that is found below the sloping updraft of
the main convective cell in the convective region. Further aspects of the pressure
perturbation field in the convective zone can be seen in the time-averaged model
results. The pressure perturbation pattern corresponding to the time-averaged
model circulation in Fig. 9.25 is shown in Fig. 9.28. The total perturbation
p* (Fig.
9.28a) again displays the small-scale low in the convective zone.
It
is the strong
minimum at the top of the cold-pool head,
just
below the sloping front-to-rear
ascent, as seen in the aircraft data composite of Fig. 9.19. In addition, there are
three maxima of
p*: a small-scale peak of p* at the surface at the leading edge of
the cold-pool head, a shallow secondary maximum of
p* in the boundary layer
directly below the cold-pool head, and a broad maximum aloft extending over the
trailing stratiform region. In Fig. 9.28b and c, the total pressure perturbation is
decomposed into its dynamic and buoyancy components
p~
and
Pb.
From these
additional cross sections, we can see the relative contribution of each component
to the total pressure perturbation field in Fig. 9.28a. Comparison of Fig. 9.28a and
b shows that the total field is dominated by the buoyancy component. The only
exception is that the small-scale peak of
p* seen at the surface at the leading edge
of the cold-pool head in Fig. 9.28a is not apparent in the buoyancy pressure-
perturbation field.
It
is entirely a dynamically produced feature, which is strongly
represented in Fig. 9.28c. This feature was described in Sec. 8.9 [Eq. (8.43)] as a
dynamically produced feature that occurs where the low-level inflow current is
stagnated and kinetic energy is converted to enthalpy. This interpretation is con-
firmed by its appearance only in the field of
Pb.
Another feature of interest in the
Pb
field is the pressure minimum that occurs in the middle of the cold-pool head.
This minimum is associated with the vorticity centered at this location in the
interface of the overlying rearward flow and the underlying rear-to-front flow in
the cold pool. A vortex is centered at this point in the mean flow pattern shown in
Fig. 9.25b. Such a vortex and an accompanying cyclostrophic pressure minimum
were seen to be characteristic of a density-current outflow head in Sec. 8.9
[Eq,
(8.44)]. From Fig. 9.28b and c, it is evident that this minimum of the dynamic
pressure perturbation component lies
just
forward of the minimum in the buoy-
ancy component, which is an effect of the deep layer of buoyant updraft air lying
above this point. The superposition of the two minima leads to the broader,
stronger minimum in the total field (Fig. 9.28a) than would be present from the
effect of buoyancy alone.
It
is thus an oversimplification to think of the pressure
minimum seen in data such as those in Fig. 9.19 as being either purely a hydro-
static or a purely dynamic pressure minimum.
9.2.2.8 Influence
of
the Stratiform Region on the
Convective Region
The foregoing observations and model results have led to the conceptualization
of the convective portion of the squall line with trailing stratiform precipitation in
Fig. 9.29. The low-e, (dry) rear inflow air enters the convective region from the
rear and descends into the cold-pool head, after passing through the trailing strati-
form precipitation
just
below the base of the trailing stratiform cloud deck. A
second source of low-s, air for the cold-pool head is the transient downdraft