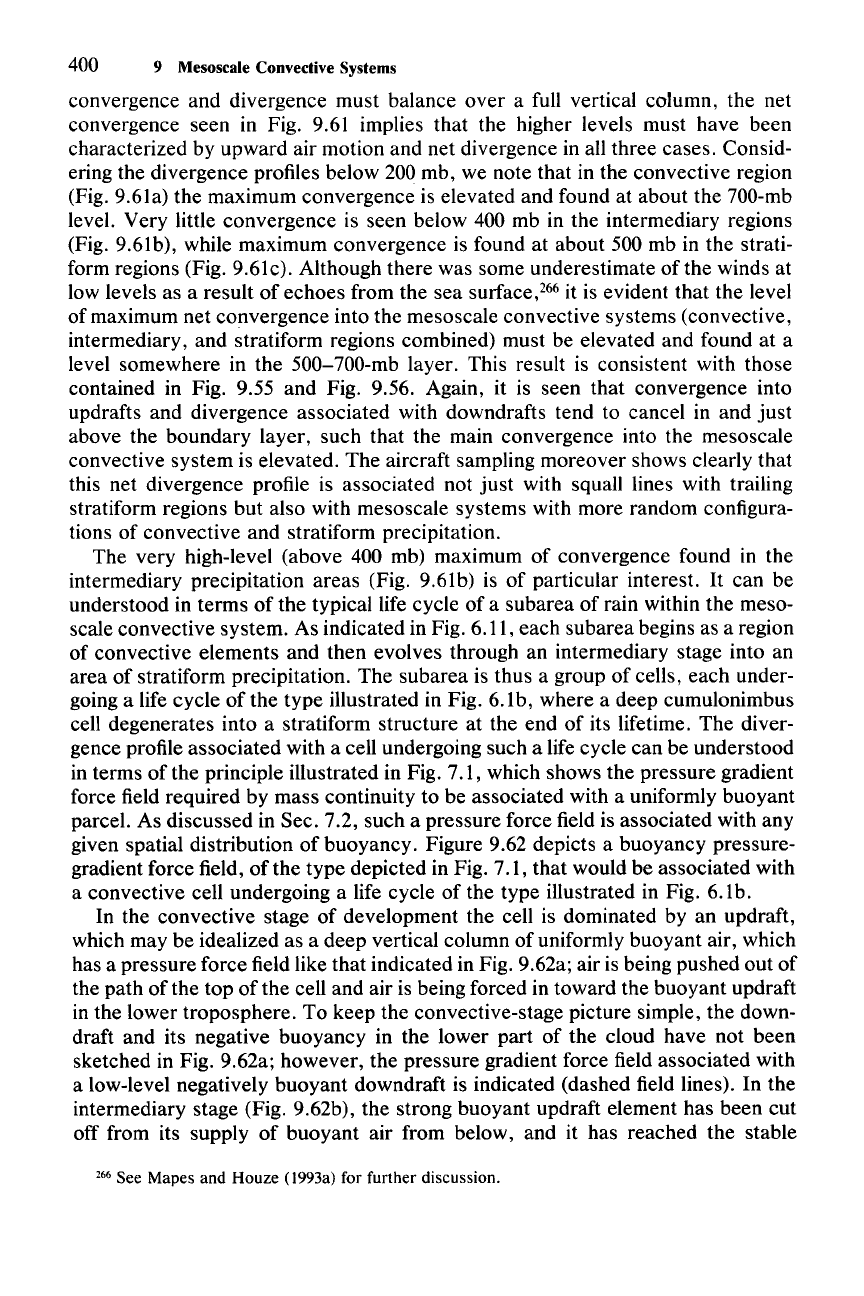
400 9 Mesoscale Convective Systems
convergence and divergence must balance over a full vertical column, the net
convergence seen in Fig. 9.61 implies that the higher levels must have been
characterized by upward air motion and net divergence in all three cases. Consid-
ering the divergence profiles below 200 mb, we note that in the convective region
(Fig. 9.6Ia) the maximum convergence is elevated and found at about the 700-mb
level. Very little convergence is seen below 400 mb in the intermediary regions
(Fig. 9.6Ib), while maximum convergence is found at about 500 mb in the strati-
form regions (Fig. 9.6Ic). Although there was some underestimate of the winds at
low levels as a result
of
echoes from the sea surface.P" it is evident that the level
of maximum net convergence into the mesoscale convective systems (convective,
intermediary, and stratiform regions combined) must be elevated and found at a
level somewhere in the 500-700-mb layer. This result is consistent with those
contained in Fig. 9.55 and Fig. 9.56. Again, it is seen that convergence into
updrafts and divergence associated with downdrafts tend to cancel in and
just
above the boundary layer, such that the main convergence into the mesoscale
convective system is elevated. The aircraft sampling moreover shows clearly that
this net divergence profile is associated not
just
with squall lines with trailing
stratiform regions but also with mesoscale systems with more random configura-
tions of convective and stratiform precipitation.
The very high-level (above 400 mb) maximum of convergence found in the
intermediary precipitation areas (Fig. 9.6Ib) is of particular interest. It can be
understood in terms
of
the typical life cycle of a subarea of rain within the meso-
scale convective system. As indicated in Fig. 6.11, each subarea begins as a region
of convective elements and then evolves through an intermediary stage into an
area of stratiform precipitation. The subarea is thus a group of cells, each under-
going a life cycle of the type illustrated in Fig.
6.lb,
where a deep cumulonimbus
cell degenerates into a stratiform structure at the end of its lifetime. The diver-
gence profile associated with a cell undergoing such a life cycle can be understood
in terms
of
the principle illustrated in Fig. 7.1, which shows the pressure gradient
force field required by mass continuity to be associated with a uniformly buoyant
parcel. As discussed in Sec. 7.2, such a pressure force field is associated with any
given spatial distribution
of
buoyancy. Figure 9.62 depicts a buoyancy pressure-
gradient force field, of the type depicted in Fig. 7.1, that would be associated with
a convective cell undergoing a life cycle of the type illustrated in Fig.
6.lb.
In the convective stage of development the cell is dominated by an updraft,
which may be idealized as a deep vertical column of uniformly buoyant air, which
has a pressure force field like that indicated in Fig. 9.62a; air is being pushed out of
the path of the top of the cell and air is being forced in toward the buoyant updraft
in the lower troposphere. To keep the convective-stage picture simple, the down-
draft and its negative buoyancy in the lower part of the cloud have not been
sketched in Fig. 9.62a; however, the pressure gradient force field associated with
a low-level negatively buoyant downdraft is indicated (dashed field lines). In the
intermediary stage (Fig. 9.62b), the strong buoyant updraft element has been cut
off from its supply
of
buoyant air from below, and it has reached the stable
266 See Mapes and Houze (I993a) for further discussion.