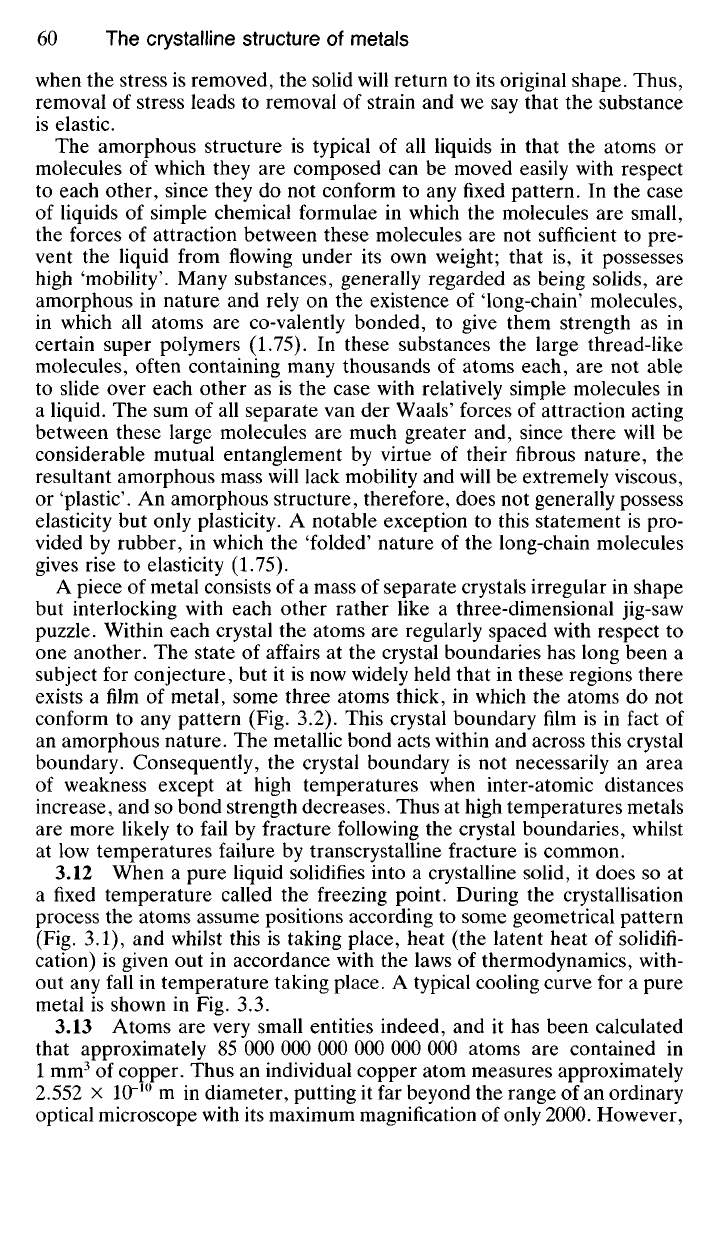
when the stress is removed, the solid will return to its original shape. Thus,
removal of stress leads to removal of strain and we say that the substance
is elastic.
The amorphous structure is typical of all liquids in that the atoms or
molecules of which they are composed can be moved easily with respect
to each other, since they do not conform to any fixed pattern. In the case
of liquids of simple chemical formulae in which the molecules are small,
the forces of attraction between these molecules are not sufficient to pre-
vent the liquid from flowing under its own weight; that is, it possesses
high 'mobility'. Many substances, generally regarded as being solids, are
amorphous in nature and rely on the existence of 'long-chain' molecules,
in which all atoms are co-valently bonded, to give them strength as in
certain super polymers (1.75). In these substances the large thread-like
molecules, often containing many thousands of atoms each, are not able
to slide over each other as is the case with relatively simple molecules in
a liquid. The sum of all separate van der Waals' forces of attraction acting
between these large molecules are much greater and, since there will be
considerable mutual entanglement by virtue of their fibrous nature, the
resultant amorphous mass will lack mobility and will be extremely viscous,
or 'plastic'. An amorphous structure, therefore, does not generally possess
elasticity but only plasticity. A notable exception to this statement is pro-
vided by rubber, in which the 'folded' nature of the long-chain molecules
gives rise to elasticity (1.75).
A piece of metal consists of a mass of separate crystals irregular in shape
but interlocking with each other rather like a three-dimensional jig-saw
puzzle. Within each crystal the atoms are regularly spaced with respect to
one another. The state of affairs at the crystal boundaries has long been a
subject for conjecture, but it is now widely held that in these regions there
exists a film of metal, some three atoms thick, in which the atoms do not
conform to any pattern (Fig. 3.2). This crystal boundary film is in fact of
an amorphous nature. The metallic bond acts within and across this crystal
boundary. Consequently, the crystal boundary is not necessarily an area
of weakness except at high temperatures when inter-atomic distances
increase, and so bond strength decreases. Thus at high temperatures metals
are more likely to fail by fracture following the crystal boundaries, whilst
at low temperatures failure by transcrystalline fracture is common.
3.12 When a pure liquid solidifies into a crystalline solid, it does so at
a fixed temperature called the freezing point. During the crystallisation
process the atoms assume positions according to some geometrical pattern
(Fig. 3.1), and whilst this is taking place, heat (the latent heat of solidifi-
cation) is given out in accordance with the laws of thermodynamics, with-
out any fall in temperature taking place. A typical cooling curve for a pure
metal is shown in Fig. 3.3.
3.13 Atoms are very small entities indeed, and it has been calculated
that approximately 85 000 000 000 000 000 000 atoms are contained in
1 mm
3
of copper. Thus an individual copper atom measures approximately
2.552 x 10~
10
m in diameter, putting it far beyond the range of an ordinary
optical microscope with its maximum magnification of only 2000. However,