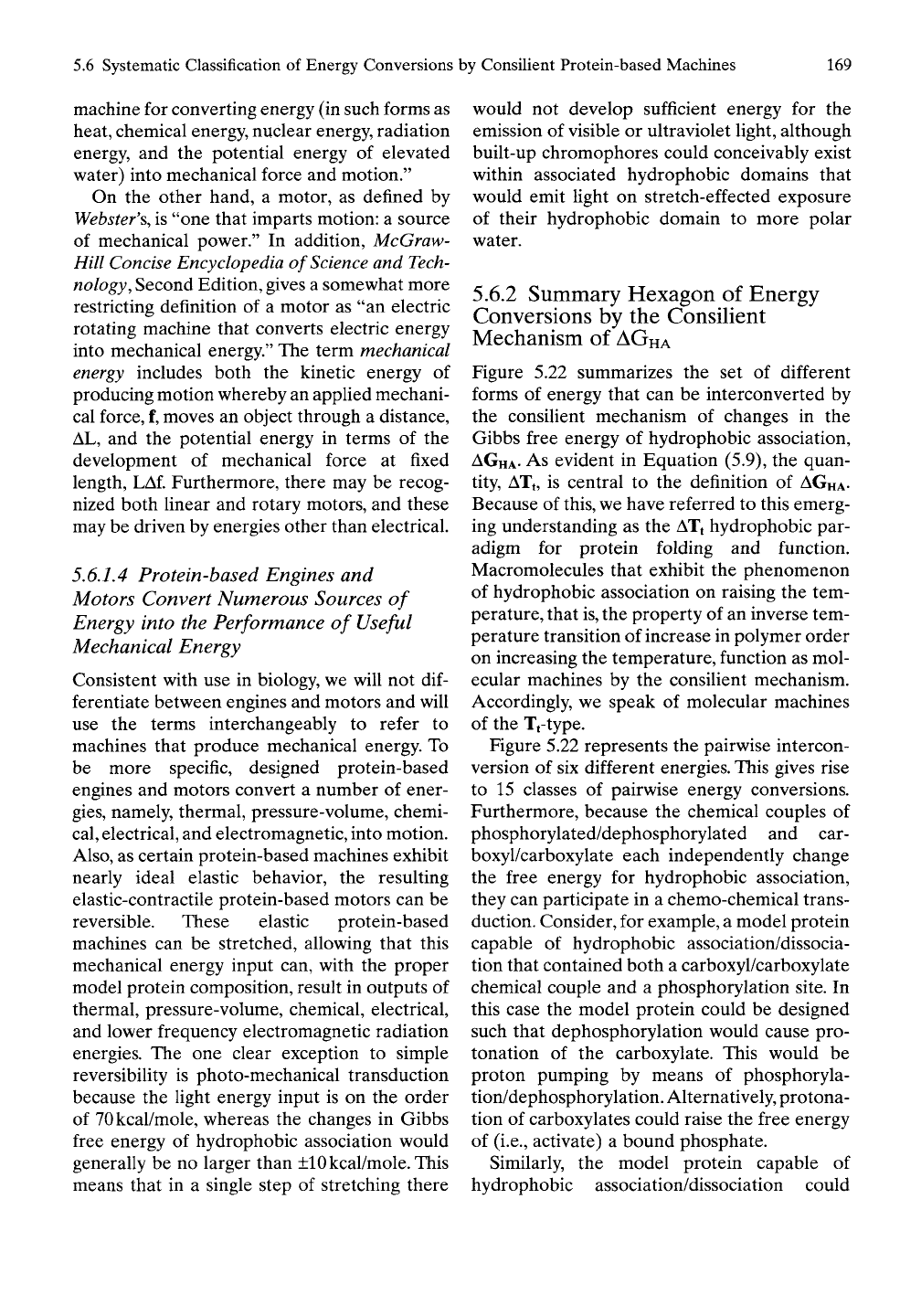
5.6 Systematic Classification of Energy Conversions by Consilient Protein-based Machines
169
machine for converting energy (in such forms as
heat, chemical energy, nuclear energy, radiation
energy, and the potential energy of elevated
water) into mechanical force and motion."
On the other hand, a motor, as defined by
Webster's, is "one that imparts motion: a source
of mechanical power." In addition, McGraw-
Hill Concise Encyclopedia of Science and Tech-
nology, Second Edition, gives a somewhat more
restricting definition of a motor as "an electric
rotating machine that converts electric energy
into mechanical energy." The term mechanical
energy includes both the kinetic energy of
producing motion whereby an applied mechani-
cal force, f, moves an object through a distance,
AL,
and the potential energy in terms of the
development of mechanical force at fixed
length, LAf. Furthermore, there may be recog-
nized both linear and rotary motors, and these
may be driven by energies other than electrical.
5.6.1.4 Protein-based Engines and
Motors Convert Numerous Sources of
Energy into the Performance of Useful
Mechanical Energy
Consistent with use in biology, we will not
dif-
ferentiate between engines and motors and will
use the terms interchangeably to refer to
machines that produce mechanical energy. To
be more specific, designed protein-based
engines and motors convert a number of ener-
gies,
namely, thermal, pressure-volume, chemi-
cal,
electrical, and electromagnetic, into motion.
Also,
as certain protein-based machines exhibit
nearly ideal elastic behavior, the resulting
elastic-contractile protein-based motors can be
reversible. These elastic protein-based
machines can be stretched, allowing that this
mechanical energy input can, with the proper
model protein composition, result in outputs of
thermal, pressure-volume, chemical, electrical,
and lower frequency electromagnetic radiation
energies. The one clear exception to simple
reversibility is photo-mechanical transduction
because the hght energy input is on the order
of 70kcal/mole, whereas the changes in Gibbs
free energy of hydrophobic association would
generally be no larger than ±10kcal/mole. This
means that in a single step of stretching there
would not develop sufficient energy for the
emission of visible or ultraviolet light, although
built-up chromophores could conceivably exist
within associated hydrophobic domains that
would emit light on stretch-effected exposure
of their hydrophobic domain to more polar
water.
5.6.2 Summary Hexagon of Energy
Conversions by the Consilient
Mechanism of
AGHA
Figure 5.22 summarizes the set of different
forms of energy that can be interconverted by
the consilient mechanism of changes in the
Gibbs free energy of hydrophobic association,
AGHA- AS
evident in Equation (5.9), the quan-
tity, ATt, is central to the definition of
AGHA-
Because of this, we have referred to this emerg-
ing understanding as the ATt hydrophobic par-
adigm for protein folding and function.
Macromolecules that exhibit the phenomenon
of hydrophobic association on raising the tem-
perature, that
is,
the property of an inverse tem-
perature transition of increase in polymer order
on increasing the temperature, function as mol-
ecular machines by the consilient mechanism.
Accordingly, we speak of molecular machines
of the Tt-type.
Figure 5.22 represents the pairwise intercon-
version of six different energies. This gives rise
to 15 classes of pairwise energy conversions.
Furthermore, because the chemical couples of
phosphorylated/dephosphorylated and car-
boxyl/carboxylate each independently change
the free energy for hydrophobic association,
they can participate in a chemo-chemical trans-
duction. Consider, for example, a model protein
capable of hydrophobic association/dissocia-
tion that contained both a carboxyl/carboxylate
chemical couple and a phosphorylation site. In
this case the model protein could be designed
such that dephosphorylation would cause pro-
tonation of the carboxylate. This would be
proton pumping by means of phosphoryla-
tion/dephosphorylation. Alternatively, protona-
tion of carboxylates could raise the free energy
of (i.e., activate) a bound phosphate.
Similarly, the model protein capable of
hydrophobic association/dissociation could