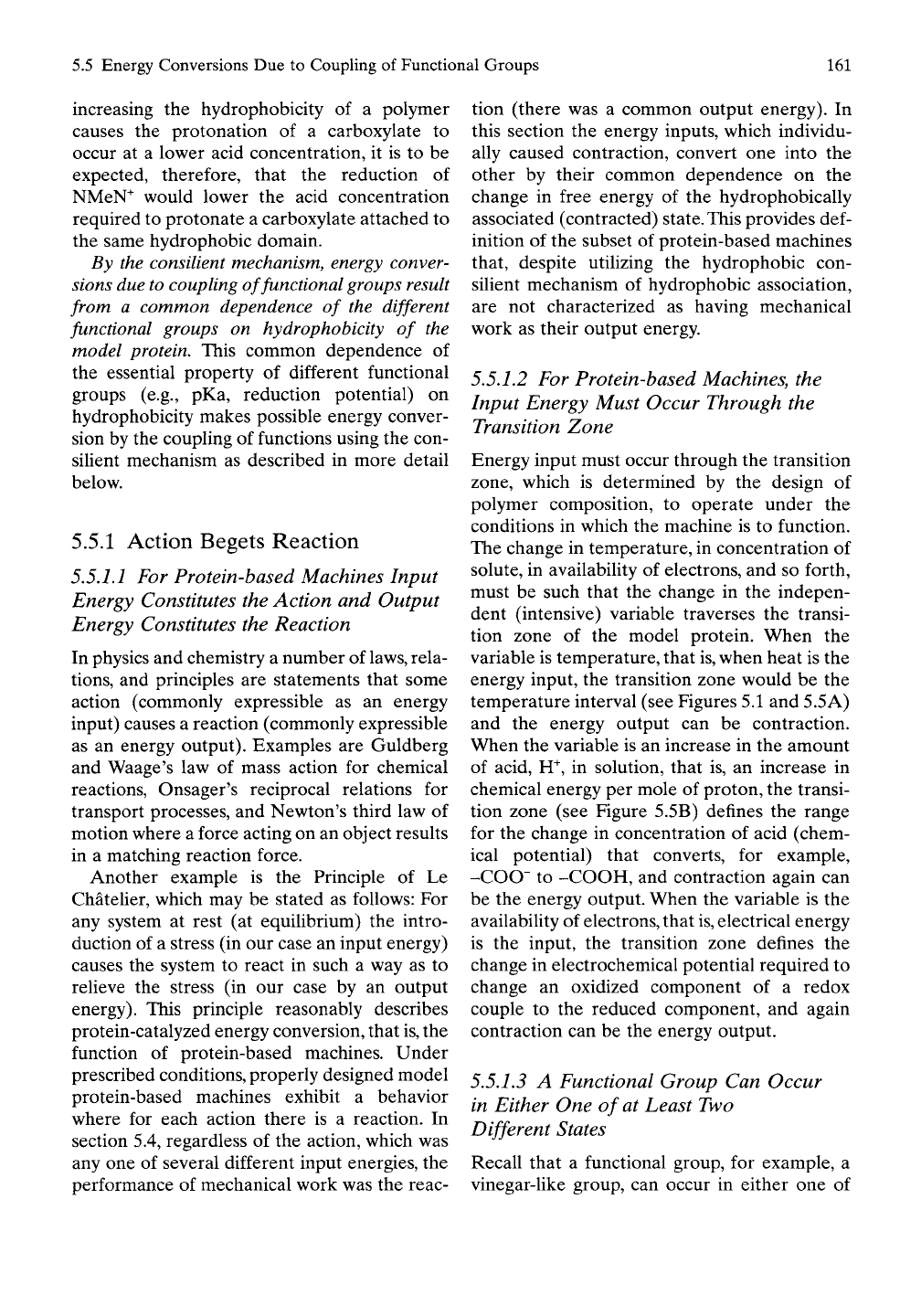
5.5 Energy Conversions Due to Coupling of Functional Groups
161
increasing the hydrophobicity of a polymer
causes the protonation of a carboxylate to
occur at a lower acid concentration, it is to be
expected, therefore, that the reduction of
NMeN^ would lower the acid concentration
required to protonate a carboxylate attached to
the same hydrophobic domain.
By the consilient mechanism, energy conver-
sions due to coupling of functional groups result
from a common dependence of the different
functional groups on hydrophobicity of the
model protein. This common dependence of
the essential property of different functional
groups (e.g., pKa, reduction potential) on
hydrophobicity makes possible energy conver-
sion by the coupling of functions using the con-
silient mechanism as described in more detail
below.
5.5.1 Action Begets Reaction
5.5.1.1 For Protein-based Machines Input
Energy Constitutes the Action and Output
Energy Constitutes the Reaction
In physics and chemistry a number of laws, rela-
tions,
and principles are statements that some
action (commonly expressible as an energy
input) causes a reaction (commonly expressible
as an energy output). Examples are Guldberg
and Waage's law of mass action for chemical
reactions, Onsager's reciprocal relations for
transport processes, and Newton's third law of
motion where a force acting on an object results
in a matching reaction force.
Another example is the Principle of Le
ChateUer, which may be stated as follows: For
any system at rest (at equilibrium) the intro-
duction of a stress (in our case an input energy)
causes the system to react in such a way as to
relieve the stress (in our case by an output
energy). This principle reasonably describes
protein-catalyzed energy conversion, that
is,
the
function of protein-based machines. Under
prescribed conditions, properly designed model
protein-based machines exhibit a behavior
where for each action there is a reaction. In
section 5.4, regardless of the action, which was
any one of several different input energies, the
performance of mechanical work was the reac-
tion (there was a common output energy). In
this section the energy inputs, which individu-
ally caused contraction, convert one into the
other by their common dependence on the
change in free energy of the hydrophobically
associated (contracted)
state.
This provides
def-
inition of the subset of protein-based machines
that, despite utilizing the hydrophobic con-
silient mechanism of hydrophobic association,
are not characterized as having mechanical
work as their output energy.
5.5.1.2 For Protein-based Machines, the
Input Energy Must Occur Through the
Transition Zone
Energy input must occur through the transition
zone,
which is determined by the design of
polymer composition, to operate under the
conditions in which the machine is to function.
The change in temperature, in concentration of
solute, in availability of electrons, and so forth,
must be such that the change in the indepen-
dent (intensive) variable traverses the transi-
tion zone of the model protein. When the
variable is temperature, that
is,
when heat is the
energy input, the transition zone would be the
temperature interval (see Figures 5.1 and 5.5A)
and the energy output can be contraction.
When the variable is an increase in the amount
of acid, H^, in solution, that is, an increase in
chemical energy per mole of proton, the transi-
tion zone (see Figure 5.5B) defines the range
for the change in concentration of acid (chem-
ical potential) that converts, for example,
-COO"
to -COOH, and contraction again can
be the energy output. When the variable is the
availabihty of electrons, that
is,
electrical energy
is the input, the transition zone defines the
change in electrochemical potential required to
change an oxidized component of a redox
couple to the reduced component, and again
contraction can be the energy output.
5.5.1.3 A Functional Group Can Occur
in Either One of at Least Two
Different States
Recall that a functional group, for example, a
vinegar-like group, can occur in either one of