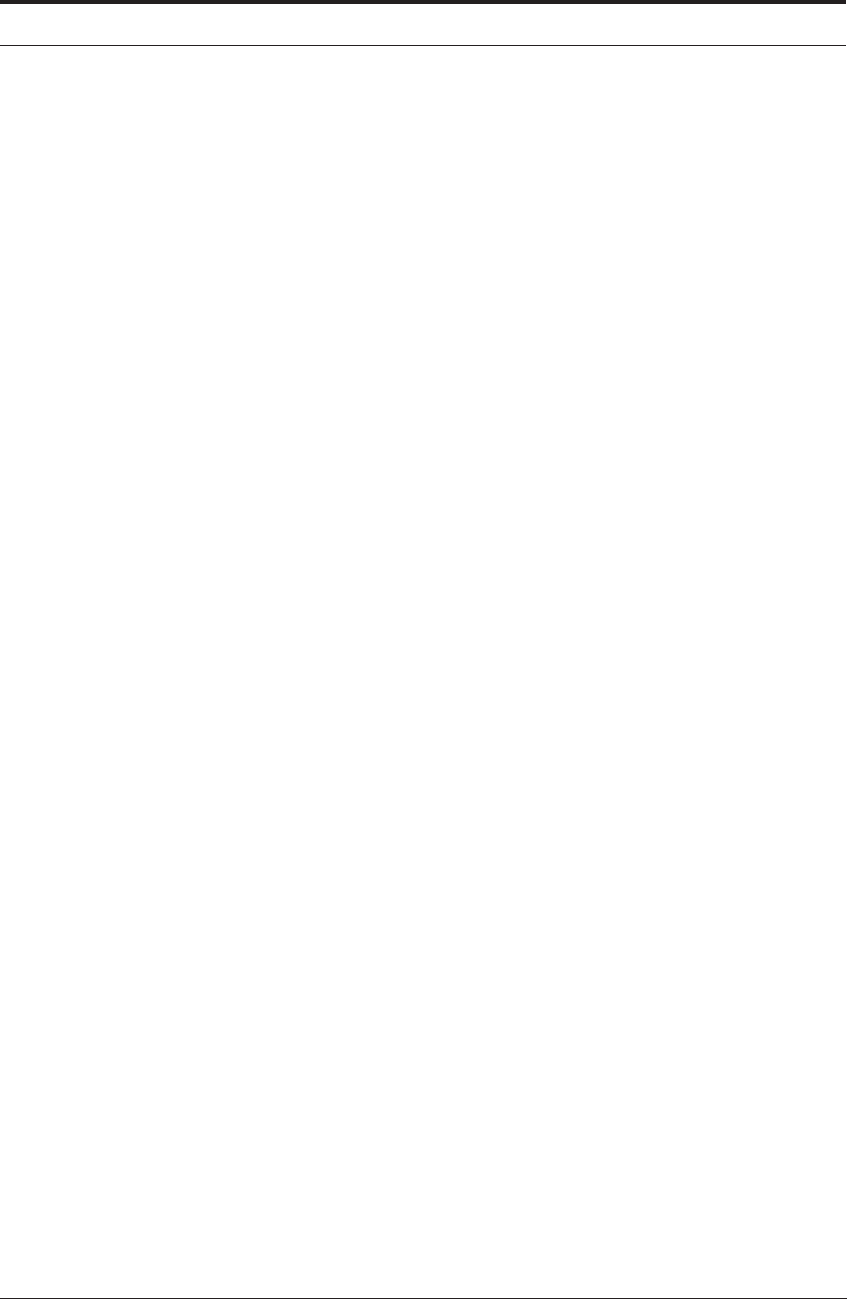
264 MACHINABILITY
Seizure between the brass and the tool seems largely to be eliminated by the lead which is con-
centrated at the interface. Figure 9.9a shows the contact area on the rake face of a high speed
steel tool after a quick-stop during cutting a leaded brass at 180 m min
-1
(600 ft/min). A concen-
tration of lead can be seen, which had been molten during cutting and had wetted the steel tool.
Confirmation of this action comes from observations of monatomic layers of lead on the under
surface of leaded brass chips. In addition, there are thicker layers, in striations, in the cutting
direction.
5
Figure 9.9 also shows the results of Wolfenden and Wright
6
using the equations in Chapter 5.
These calculations confirm that the lead is molten at the end of the chip tool contact area. This
concentration of lead at the interface reduces the tool forces. It also facilitates chip fracture by
reducing the compressive stress acting on the shear plane. Molten lead accumulates at the inter-
face because it wets the steel tool, as shown by the small contact angle of the lead droplets on the
tool. This also demonstrates that the tool surface at the interface is freed from oxide and other
surface films by the action of the work material. Lead does not wet oxidized steel surfaces.
Another result of the action of lead is a large reduction in the energy of cutting both on the
shear plane and at the tool/work interface. This results in a considerable reduction of tool tem-
perature, Figure 9.6. The effect of lead addition to 60/40 brass is to reduce the tool temperatures
below those for high conductivity copper. The lower temperatures reduce tool wear. The main
advantages are the elimination of chip control problems and the reduction in tool forces.
9.4.4 Environmental concerns
Unfortunately for “machinability” alone, lead has become recognized as a serious health haz-
ard. Possible dangers arise both in melting and machining of leaded brass and also in the use of
leaded brass as fittings for water supply. With the possibility of regulations restricting the use of
leaded brass, producers now have to consider the machining of unleaded 60/40 brass on auto-
matic machines. Continuous brass chips can be broken into short lengths by forming grooves
parallel with the cutting edge on the rake face of tools. It has been shown that certain shapes of
groove are successful in breaking chips of unleaded 60/40 brass.
7
Additions are made also to high conductivity copper to improve its machinability. Additives
have to be confined to those which do not appreciably reduce electrical conductivity, or cause
fracture during hot working. About 0.3% sulfur is usually added. This forms plastic non-metallic
inclusions of Cu
2
S. The effect is to reduce greatly the tool forces, particularly at low speeds,
Figure 9.8. Also it produces thin chips which curl and fracture readily. The surface finish is
greatly improved. The action of Cu
2
S can probably be attributed to the reduction of seizure
between copper and the rake face. The sulfide particles are plastically deformed in chip forma-
tion, and very thin layers of sulfide are observed on the contact area after quick-stops. The flow-
zone, normally present after cutting high conductivity copper, is eliminated. The essential fea-
tures of Cu
2
S as a free-machining phase are: i) its plasticity during deformation in cutting, and
ii) its strong adhesion to the tool surface, which prevents it from being swept away. One result of
the sulfur addition is a large reduction in tool temperature up to very high cutting speed (Figure
9.6). The temperatures are even lower than those when cutting leaded brass.