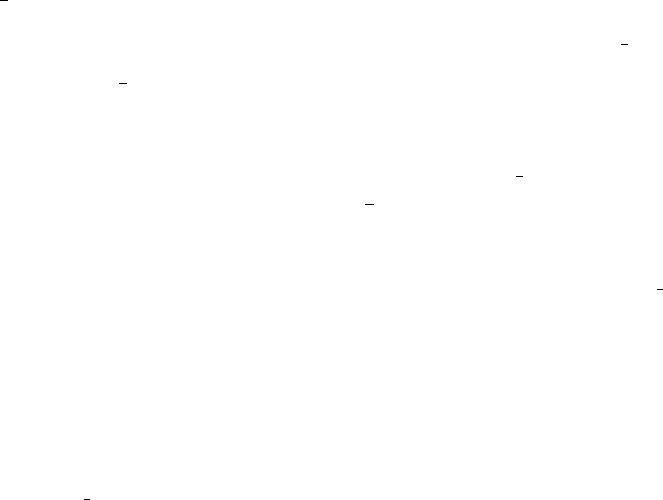
Ionization Chamber Dosimetry 165
is made of only a single material that is identically matched
by the phantom medium, P
wall
equals unity. However, the
construction of plane-parallel chambers usually employs
more than one material. Thus, the phantom medium should
be selected to match whichever material is the most impor-
tant contributor of the secondary electrons that produce the
measured ionization.
If the chamber has a very thin front wall (for which
is nearly zero), its influence can be ignored because
practically all of the electrons then originate in the phan-
tom medium. That medium should be selected to match
the principal material of which the thicker rear wall is
constructed. Since secondary electrons are projected pref-
erentially in the forward hemisphere by
60
Co gamma-ray
interactions, the electron backscattering ability of the rear
wall (a strong function of atomic number) will be the most
important influence on the ionization. If the chamber has
a thicker front wall, for
which is significantly greater
than zero, the phantom medium should be made of that
same material. However, if the back wall differs apprecia-
bly in atomic number, this in-phantom procedure should
not be expected to yield a satisfactory result, due to the
difference in electron backscattering from the back wall
in comparison with the phantom medium.
Once has been calculated, the determination of
absorbed dose for the user’s beam will proceed according
to TG-21. Since these chambers are designed primarily
for use with electron beams, calibration of those beams
only will be presented. When the chamber is placed in a
suitable phantom (medium), the dose to the medium will
be given by
(3.158)
where M is the electrometer reading (Coulombs or scale
division corrected to 22°C, 760 mm Hg). is the
ratio of the mean, restricted collision mass stopping power
of the phantom material to that of the chamber gas (ambi-
ent air). P
ion
is the factor that corrects for ionization recom-
bination losses that occur at the time of calibration of the
user’s electron beam.
In the 1983 AAPM protocol, the replacement correc-
tion factor P
repl
is taken as unity for plane-parallel cham-
bers, irrespective of electron-beam energy. Two of the
commercial chambers (Capintec and Markus) show clear
experimental evidence that P
repl
decreases with decreasing
electron energy. The Netherlands Commission on Radia-
tion Dosimetry, in their Code of Practice of the Dosimetry
of High-Energy Electron Beams, considered this situation
for the Markus chambers and recommended the following
equation for P
repl
(designated p
f
in their protocol):
(3.159)
For five commonly used parallel-plate ion chambers,
Monte Carlo calculations of the wall attenuation and
scatter correction factors (
K
wall
or ) and the
correction for nonhomogeneous composition of the cham-
ber (K
comp
or related to k
m
) were presented by Rogers.
[84] The chambers were assumed to have 0.5 g/cm
2
build-up caps made of the predominant material in each
chamber. These correction factors are needed if air-
kerma calibration factors are used to deduce the cham-
ber’s cavity-gas calibration factor, N
gas
. The scatter from
the material around the cavity more than compensates
for the attenuation in the front wall and, hence, K
wall
values are less than unity. Thin collectors or insulators
behind the collecting volume can have a major effect
because electron backscattering depends strongly on
material.
The AAPM’s TG-21 dosimetry protocol recommends
calibrating parallel-plate ion chambers by establishing the
dose in a high-energy electron beam using a calibrated
cylindrical ion chamber and then using this dose to estab-
lish the calibration of the parallel-plate chamber. By equat-
ing the dose measured at
d
max
in an electron beam using
cylindrical and parallel-plate ion chambers, one finds by
following TG-21 that
(3.160)
where the charge readings from ion chambers are repre-
sented by
M and are understood to be corrected for tem-
perature, pressure, ion recombination, and polarity effects.
If an electron beam is not to be used, there are two
options: base the calibration on an in-air air-kerma cali-
bration factor, , or base
the calibration on an in-phantom absorbed-dose calibra-
tion factor, N
D
, or equivalent.
If the in-air route is chosen, N
gas
is determined from
(3.161)
where N
K
is the air-kerma calibration factor, is the
fraction of the electron’s energy lost by radiative pro-
cesses, K
wall
( ) corrects for the lack of charged-
particle equilibrium in the chamber walls (caused mostly
by photon attenuation and scatter), corrects for any
charge-collection losses in the calibration beam, and K
comp
corrects for any components in the chamber made of mate-
rials different from the wall material.
If the calibration of the parallel-plate chamber is done
starting from an absorbed-dose measurement in a phantom,
N
gas
pp
D
med
MN
gas
pp
L/
()
gas
med
P
ion
P
repl
L/
()
gas
med
P
repl
1 0.041e
0.4E
z
A
wall
1
K
att
1
K
wall
N
gas cyl,
M
cyl
P
repl
cyl
P
wall
cyl
M
pp
P
repl
pp
P
wall
pp
------------------------------
Gy/C()
N
K
N
K
N
X
W/e
air
/1
g
()()[]
N
gas
N
K
1 g()
L/
()
air
wall
en
/
()
wall
air
K
wall
K
comp
K
ion
c
-------------------------------------------------------------------------------
GyC
1
()
g
A
wall
1
K
ion
c
Ch-03.fm(part 2) Page 165 Friday, November 10, 2000 11:59 AM