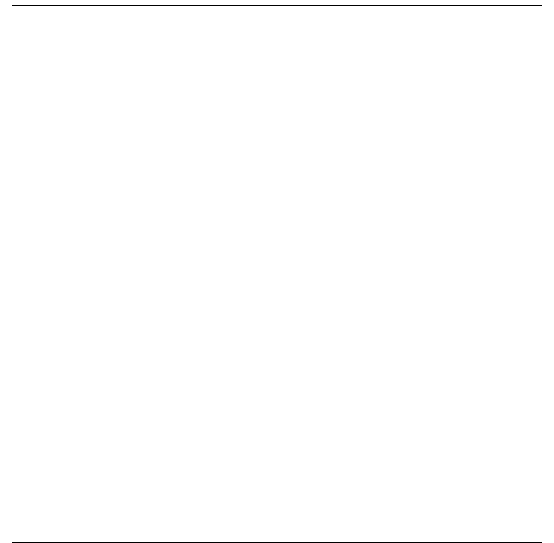
144 Radiation Dosimetry: Instrumentation and Methods
is the energy deposited by electrons generated by the
ith primary photon interaction, is the energy deposited
by electrons generated from the second- and higher-order
scattered photons that arise from the ith primary photon,
and d
i
is the number of mean free paths in the chamber
to the point of interaction of the ith primary photon.
The AAPM protocol (TG39) includes a cavity replace-
ment factor p
repl
that differs from unity for some chambers
but assumes that the wall perturbation factor, p
wall
, may
be taken as unity. The perturbation of the wall has been
determined by Nilsson et al. [60], using a large plane-
parallel ionization chamber with exchangeable front and
back walls. The results show that in many commercial
chambers there is an energy-dependent p
wall
factor, mainly
due to differences in backscatter from the often thick
chamber body as compared to the phantom material.
Backscatter in common phantom and chamber materials
may differ by as much as 2% at low electron energies.
The front walls are often thin, resulting in negligible per-
turbation, but the 0.5-mm front wall of graphite in the
NACP chamber was found to increase the response by
0.7% in a PMMA phantom.
The BPPC-1 chamber has a negligible polarity effect.
Thus, all measurements were made with the same polarity
using a field strength of 50 V mm
1
. The leakage current
was less than 0.01 pA, which is less than 0.01% of the
ionization current obtained in the measurements.
Plane-parallel chambers commercially available often
have composite backscatter walls with thin electrodes of
a material different from the chamber body. Nilsson et al.
measurements simulating the NACP (Scanditronix
NACP-02) and the Attix chambers (Gammex/RMI
model 449) were made both with and without the com-
posite electrodes in a PMMA phantom. The Roos chamber
(PTW type 34001 or Wellhofer IC40), with walls made
of PMMA, was simulated by just using the BPPC-1 cham-
ber in the phantom, and the ionization obtained with this
combination was used as a reference. The measuring
geometries of the different simulated chambers are indi-
cated in Figure 3.56.
If measurements are not performed in a PMMA phan-
tom, the 1-mm front and back walls of PMMA in the Roos
chamber may have an effect on the response of the cham-
ber. Some measurements were thus performed simulating
the Roos chamber in a phantom of polystyrene and com-
pared to the use of a homogeneous polystyrene chamber
in a polystyrene phantom. The experiments were simu-
lated using the EGS4 Monte Carlo system.
Calculations were performed assuming a monoener-
getic parallel electron beam with an energy equal to the
mean energy at the surface obtained from depth-dose dis-
tributions in water and calculated according to IAEA.
The results of the variation of the backscatter factor
with the mean electron energy at
R
100
are plotted in Figure
3.57. The data are normalized to backscatter from PMMA.
Solid Water appears to be equivalent to PMMA with
respect to backscatter within the experimental uncertain-
ties. Graphite has a backscatter factor about 0.5–0.6%
higher than PMMA, showing a slight increase with
decreasing energy. The backscatter factors of the other
materials show a stronger energy dependence. White and
clear polystyrene have similar backscatter factors, around
TABLE 3.15
Wall Materials Used in the Measurements
Composition
(Z of constituent: fraction
by weight)
Density
(kg m
–3
)
a
Material
Graphite, C C: 1.00 1.69 10
3
Polymethylmethacry-
late, PMMA
(C
5
H
8
0
2
)
n
H:0.0805, C:0.5998, O:0.3196 1.17 10
3
Polyethylene (C
2
H
4
)
n
H:0.1437, C:0.8563 0.95 10
3
Clear polystyrene
(C
8
H
8
)
n
H:0.0774, C:0.9226 1.04 10
3
White polystyrene
(C
8
H
8
)
n
+ TiO
2
1.06 10
3
Solid Water-457
TM
H:0.081, C:0.672, N:0.024,
O:0.199, Cl:0.001,
1.04 10
3
Ca:0.023
Plastic Water
TM
H:0.0926, C:0.6282, N:0.010,
O:0.1794,
1.02 10
3
Cl:0.0096, Ca:0.0795,
Br:0.0003
Source: From Reference [60]. With permission.
r
i
0
r
i
1
Ch-03.fm(part 2) Page 144 Friday, November 10, 2000 11:59 AM