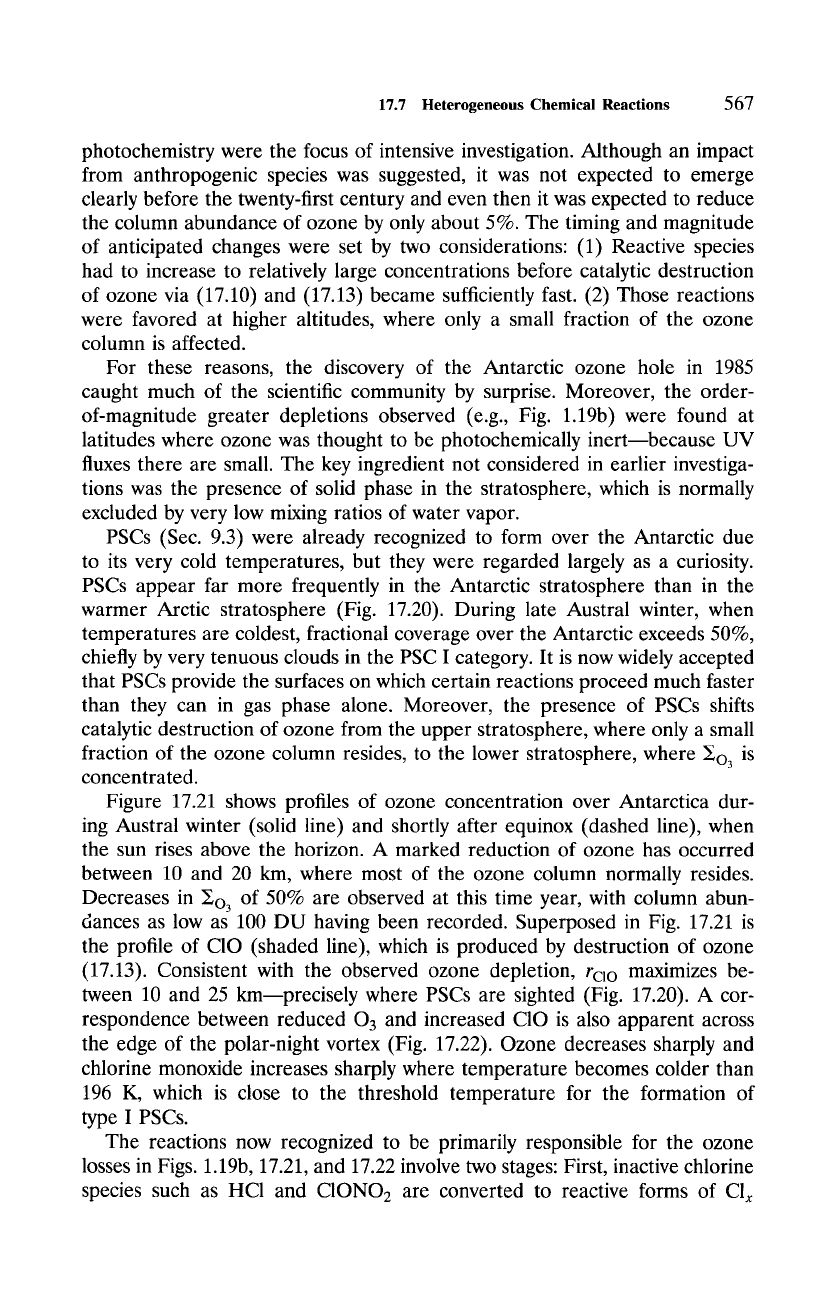
17.7 Heterogeneous Chemical Reactions
567
photochemistry were the focus of intensive investigation. Although an impact
from anthropogenic species was suggested, it was not expected to emerge
clearly before the twenty-first century and even then it was expected to reduce
the column abundance of ozone by only about 5%. The timing and magnitude
of anticipated changes were set by two considerations: (1) Reactive species
had to increase to relatively large concentrations before catalytic destruction
of ozone via (17.10) and (17.13) became sufficiently fast. (2) Those reactions
were favored at higher altitudes, where only a small fraction of the ozone
column is affected.
For these reasons, the discovery of the Antarctic ozone hole in 1985
caught much of the scientific community by surprise. Moreover, the order-
of-magnitude greater depletions observed (e.g., Fig. 1.19b) were found at
latitudes where ozone was thought to be photochemically inert~because UV
fluxes there are small. The key ingredient not considered in earlier investiga-
tions was the presence of solid phase in the stratosphere, which is normally
excluded by very low mixing ratios of water vapor.
PSCs (Sec. 9.3) were already recognized to form over the Antarctic due
to its very cold temperatures, but they were regarded largely as a curiosity.
PSCs appear far more frequently in the Antarctic stratosphere than in the
warmer Arctic stratosphere (Fig. 17.20). During late Austral winter, when
temperatures are coldest, fractional coverage over the Antarctic exceeds 50%,
chiefly by very tenuous clouds in the PSC I category. It is now widely accepted
that PSCs provide the surfaces on which certain reactions proceed much faster
than they can in gas phase alone. Moreover, the presence of PSCs shifts
catalytic destruction of ozone from the upper stratosphere, where only a small
fraction of the ozone column resides, to the lower stratosphere, where Eo3 is
concentrated.
Figure 17.21 shows profiles of ozone concentration over Antarctica dur-
ing Austral winter (solid line) and shortly after equinox (dashed line), when
the sun rises above the horizon. A marked reduction of ozone has occurred
between 10 and 20 km, where most of the ozone column normally resides.
Decreases in Eo3 of 50% are observed at this time year, with column abun-
dances as low as 100 DU having been recorded. Superposed in Fig. 17.21 is
the profile of C10 (shaded line), which is produced by destruction of ozone
(17.13). Consistent with the observed ozone depletion, rcl o maximizes be-
tween 10 and 25 km~precisely where PSCs are sighted (Fig. 17.20). A cor-
respondence between reduced 03 and increased C10 is also apparent across
the edge of the polar-night vortex (Fig. 17.22). Ozone decreases sharply and
chlorine monoxide increases sharply where temperature becomes colder than
196 K, which is close to the threshold temperature for the formation of
type I PSCs.
The reactions now recognized to be primarily responsible for the ozone
losses in Figs. 1.19b, 17.21, and 17.22 involve two stages: First, inactive chlorine
species such as HC1 and C1ONO2 are converted to reactive forms of C1 x