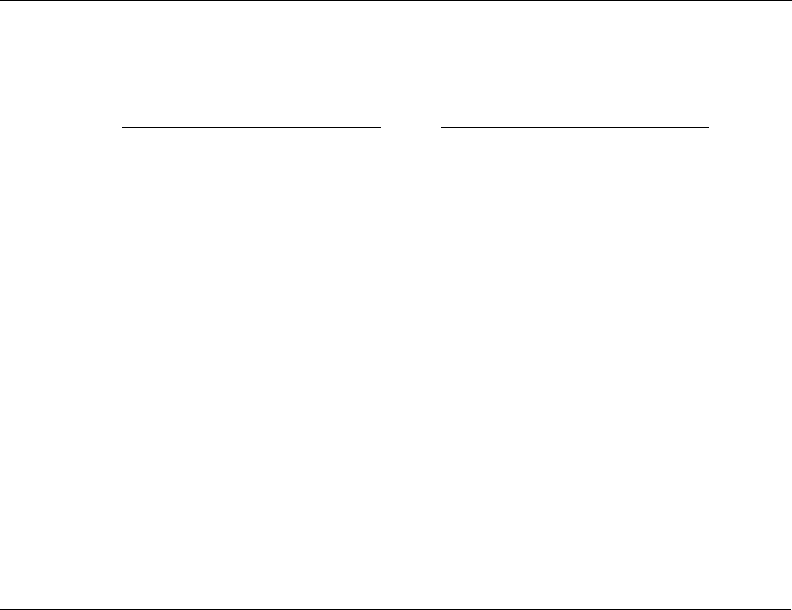
Lubricants for the Disk Drive Industry 569
motor. Recent developments on molecular dynamic simulation promise to bridge this gap between
molecular structure and thermodynamics. For example, Bair et al. [68] have demonstrated good
agreement between high shear viscosity measurements and predictions from a molecular dynam-
ics simulation. Yet, the model requires input of a particular molecular structure, in their case,
squalane, and numerically intensive computations that are required to predict the viscosity. Even
more complexity is involved if one tries to similarly predict the viscosity of a more complex polar
molecule such as diesters.
Oil blends, or viscosity-reducing additives, have also been considered. Blending oils change the
pressure–viscosity coef cient [55] and provide an intermediate viscosity [69,70]. The vaporization
energy and entropy and ow activation energy and entropy are shown for a blend of polar diester
oil in nonpolar poly alpha ole n (PAO) oil in Figure 22.31 [1]. In this case, the vaporization energy
of the blend was less than that of the pure components. The entropy of the blend was higher than
that of the pure components. The reduction in the vaporization energy outweighed the effect of the
increase in liquid entropy, and the vapor pressure of the blend was higher than that of the pure com-
ponents. There is, however, an interesting dip in the properties near 95% DOS, which was reproduc-
ible. The ow activation energy and entropy of the blends are shown in Figures 22.31c and 22.31d.
The ow activation energy is approximately given by a linear combination of the weight fractions
of blend components. While the blend viscosity is actually less than the linear combination due
to an increase in the ow activation entropy near 50%. Taking the analysis one step further, the
ow activation rotational entropy has a sharp maximum near 95% DOS in Figure 22.32a. The ratio
n = ∆E
vap
/∆E
vis
has a sharp minimum near 95% DOS (Figure 22.32b). This unusual behavior near
95% DOS suggests that synergistic effects are possible in oil blends. These probably arise from clus-
tering of the nonpolar oil with the aliphatic chains of the diester. Perhaps, this type of effect could be
exploited to develop viscosity-reducing additives that have a lower vapor pressure than the base oil.
TABLE 22.20
Evaporation and Flow Thermodynamic Properties Calculated from Vapor Pressure and
Viscosity Data for Model Fluid Dynamic Bearing Oils
Evaporation Flow
Oil
∆E
vap
(kJ/mol)
∆S
vap
(J/mol K)
S
liq
(J/mol K)
∆E
vis
(kJ/mol)
∆S
vis
(J/mol K)
∆S
vis
rot
(J/mol K) n
DBS 90.7 160 114 20.6 −4.2 −3.1 4.4
DOA 94.4 160 117 24.7 4.1 6.61 3.8
DOZ 84.5 125 154 26.7 6.8 19.8 3.2
DOS 108.6 175 105 26.7 5.8 2.6 4.1
DIA 94.1 143 137 28.9 11.2 18.1 3.3
TRIB 81.8 154 163 25.1 10.5 12.5 3.3
TRIH 93.3 156 167 23.2 −1.5 2.4 4.0
TRIO 116.3 185 143 26.2 3.5 −1.4 4.4
TMP 81.0 110 218 28.9 10.4 30.3 2.8
2,2-DPP 59.1 120 144 25.5 17.0 30.4 2.3
1,3-DPP 74.2 159 105 22.3 8.4 6.6 3.4
PAO 89.6 188 80 22.7 4.5 −4.2 3.9
PRS 78.5 166 104 22.8 4.6 2.6 3.5
SQL 82.8 124 156 32.9 21.2 36.6 2.5
Note: Evaporation properties are referenced to 100°C and atmospheric pressure (100 kPa). Flow properties are referenced
to 40°C.
Source: Adapted from Rudnick, L.R. (ed.), Synthetics, Mineral Oils, and Bio-Based Lubricants Chemistry and Technology,
Chapter 38, CRC Press, Taylor & Francis Group, Boca Raton, FL, 2006.
CRC_59645_Ch022.indd 569CRC_59645_Ch022.indd 569 3/20/2009 5:44:39 PM3/20/2009 5:44:39 PM