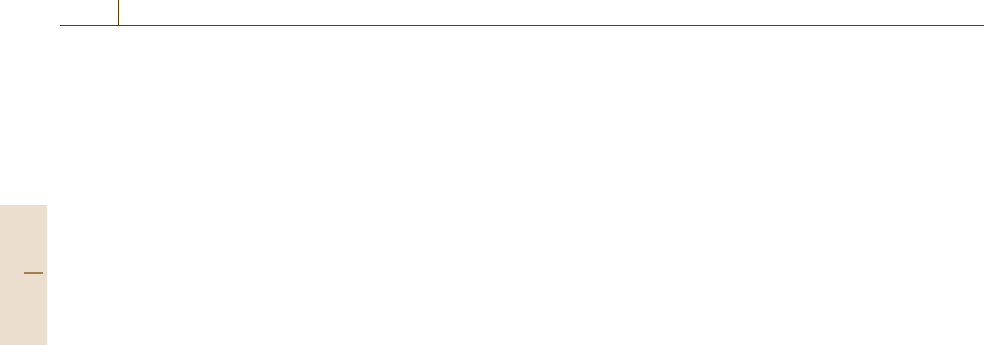
1374 Part H Automation in Medical and Healthcare Systems
76.4.1 in vitro Physiological Systems
The basic science of biomedicine often begins with cell
culture and in vitro experiments. However, a key is-
sue in translating cell-only experimental results to live
animals is the disconnect between cells in a dish and
cells interacting. One approach to this problem has
been coculturing of cells to allow cellular crosstalk
and to allow the development of three-dimensional
structure that better mimics physiology. This is a tech-
nique used in blood–brain barrier research [76.93]to
develop in vitro tests for neurotoxicity. For PK anal-
ysis, many drugs are cleared by the cytochrome P450
enzymes in the liver but cause toxicities or effects else-
where in the body. Hence, an in vitro liver cell culture
could be used to analyze drug kinetics; one such op-
tion, although the cells are encapsulated in a polymeric
coating, is described in [76.94]. A secondary concern
beyond three-dimensional (3-D) structure is spatial het-
erogeneity, which may be more difficult to recreate
in vitro [76.95].
A potential system for in vitro testing of drug PK
would be a fully integrated physiologically motivated
cell culture system: cells of different types growing in
culture, with fluid volumes for each cell type corre-
sponding to in vivo physiological volume; fluid flow
and connectivity allowing intercell crosstalk within
and between cell types (simulated physiology); rele-
vant compartments for evaluating effect, as appropriate
(e.g., tumor cells in culture for cancer PD analysis);
and the entire system automated for sensing and con-
trol. In the context of cancer drug PK and PD,the
incorporation of tumor cells would alleviate two short-
comings commonly present in current in vitro analysis:
(i) clinicallyinvalidpharmacokinetic profiles,where the
tumor cells are bathed in a constant concentration of
drug for a period of time, and (ii) cell kill analysis
based on the aforementioned pharmacokinetic profile.
While the mathematical analysis of tumor cell kill un-
der time-dependent pharmacokinetic profiles is more
complicated than the constant-concentration approach
used presently, the use of in vitro physiological systems
could serve to reduce the number of animal studies nec-
essary in drug development while also improving the
translation of in vitro results to the toxicity and efficacy
studies that have to be performed.
76.4.2 Translating in vitro to in vivo
The transition from in vitro to in vivo provides a serious
challenge to modelers and treatment designers. Phys-
iology may provide an advantage, as the connectivity
between organs in animals is well understood. Organ
weights and blood flow rates to individual organs are
available in the literature for a variety of species, in-
cluding toxicologic man [76.96]. However, this detailed
approach assumes a model of physiological structure,
which is not the generally applied practice in clini-
cal drug development. As described in Sects. 76.2.2
and 76.2.3, compartmental models are more commonly
employed in the (pre)clinical setting due to the smaller
number of parameters, reduced mathematical complex-
ity, and the relative ease of parameter estimation. In
this less detailed structure, however, the method for
incorporating in vitro information is ad hoc. Hence,
a more mechanisticapproach is desiredwhen significant
in vitro information is available because the mapping to
the in vivo situation will be improved.
As discussed above, animals are not humans.
The compartmental and population-based PK models
often developed in animals rely upon allometric scal-
ing [76.97, 98] to address the interspecies differences
in dynamic profile. While imperfect (as witnessed by
the dearth of PK-driven model-based treatment designs
deployed in the clinic), this scaling principle can pro-
vide useful insight for selecting first-in-human doses
and times to sample during phase I trials. Translat-
ing information relies to some degree on mechanistic
accuracy at the cellular level, thereby limiting the
unknown factors to the physiologically connected tis-
sues. In some cases, carefully constructed physiological
models can be successfully scaled from animals to hu-
mans by accounting for changes in physiology, such
as body fat percentage, as seen for the lipophilic an-
ticancer drug Docetaxel [76.10]. Often this requires
assumptions, such as equivalent plasma protein binding
characteristics (potentially based on binding informa-
tion from in vitro studies), and similar mechanisms of
clearance (liver metabolism, elimination in urine, etc.).
Under these assumptions, the human physiologically
based pharmacokinetic (PBPK) model (Fig.76.4) can
be successfully constructed by using the metabolic and
clearance parameters as thedegrees of freedom in fitting
human plasma data. When inconsistencies between the
scaled PBPK model and human data manifest, relaxing
the assumptions and fitting novel mechanistic behaviors
to the human plasma profile is required. The resulting
model at the human scale can provide information that
would otherwise be unattainable in humans, such as es-
timated drug concentrations in key tissues (e.g., brain)
or those prone to toxic side-effects (e.g., liver, kidney,
white blood cells).
Part H 76.4