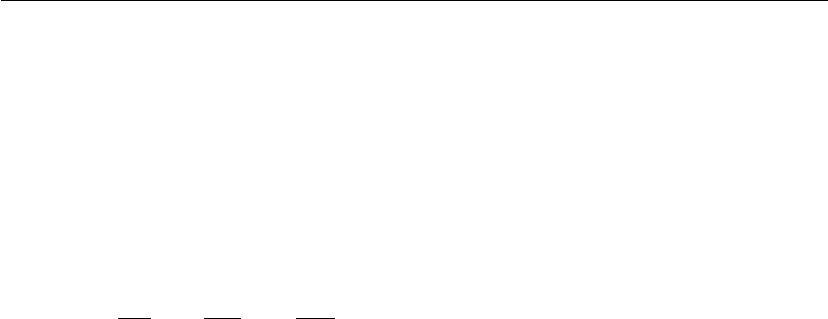
Thin Film Nucleation, Growth, and Microstructural Evolution 579
representing island size R defined as one-half the minor axis of the largest ellipse which fits
though the rough edges of the first layer island. Both R and f (R) were measured for > 100
islands at each growth temperature and R
c
determined from Eq. (12.20). R
c
results are plotted
in Figure 12.20 as a function of T
s
over the range from 650 to 1010
◦
C. The increase in the
slope at T
s
> 865
◦
C is due to an increase in the size of the smallest stable cluster, as discussed
below.
In the low temperature regime for which i* = 1, the characteristic island size R
c
is given by
[62]
R
c
=
192
π
1/6
ν
JN
s
exp
−E
s
kT
s
1/6
(12.21)
A least squares fit of the R
c
(T
s
) data in Figure 12.20 between 650 and 865
◦
C yields
E
s
= 1.4 ± 0.1 eV with ν
s
=10
13±1
s
−1
for nucleation on growing islands, in agreement with the
above results for nucleation on open terraces.
Identifying the diffusing species is fundamental to understanding atomic processes occurring
during nucleation. For TiN(001), there are several possible candidates including Ti, N, and
TiN
x
(x =1,2,3,...) admolecules. Using ab initio density functional theory (DFT), the
calculated Ti surface diffusion activation energy on TiN(001), 0.35 eV, is found to be a factor
of four smaller than the E
s
value measured by experiment [62]. Petrov et al. [67] showed that
there is a significant flux of atomic N (including N
2
+
ions which dissociate upon collision)
incident at the growing film surface during reactive magnetron sputter deposition of Ti in pure
N
2
. DFT results reveal that as N atoms diffuse and encounter each other on TiN(001), N
2
forms with a binding energy of 2.1 eV, but with an adsorption energy of only 0.2 eV with
respect to gas-phase N
2
. Thus, N
2
desorbs at near kinetic rates. Moreover, the calculated N
diffusion barrier is 0.95 eV, significantly smaller than the E
s
= 1.4 eV value obtained
experimentally. The authors therefore conclude, consistent with additional DFT calculations,
that TiN
x
(x = 1, 2, 3) admolecules are the primary diffusing species rather than Ti or N
adatoms. TiN
4
is unstable and dissociates to TiN
2
admolecules and N
2
. Under high N supply
rate conditions, as in these experiments, TiN
2
and/or TiN
3
are expected to be the primary
diffusing species, while a reduction in the N supply increases the coverage of Ti and TiN
adspecies at the expense of TiN
2
and TiN
3
leading to a change in nucleation kinetics, as
demonstrated below, by decreasing the N
2
partial pressure in an Ar/N
2
mixture.
The results in Figure 12.20 reveal a dramatic increase in the rate of change in R
c
vs T
s
at T
s
> 865
◦
C. This could be due to either a change in the nature of the primary diffusing species or
a change in the critical cluster size. Assuming that i* remains constant, the E
s
value extracted
from Figure 12.20 in the high T
s
regime is 8.4 eV, larger than all TiN
x
admolecule adsorption
energies and therefore not physically reasonable. Thus, i* must increase over the T
s
range