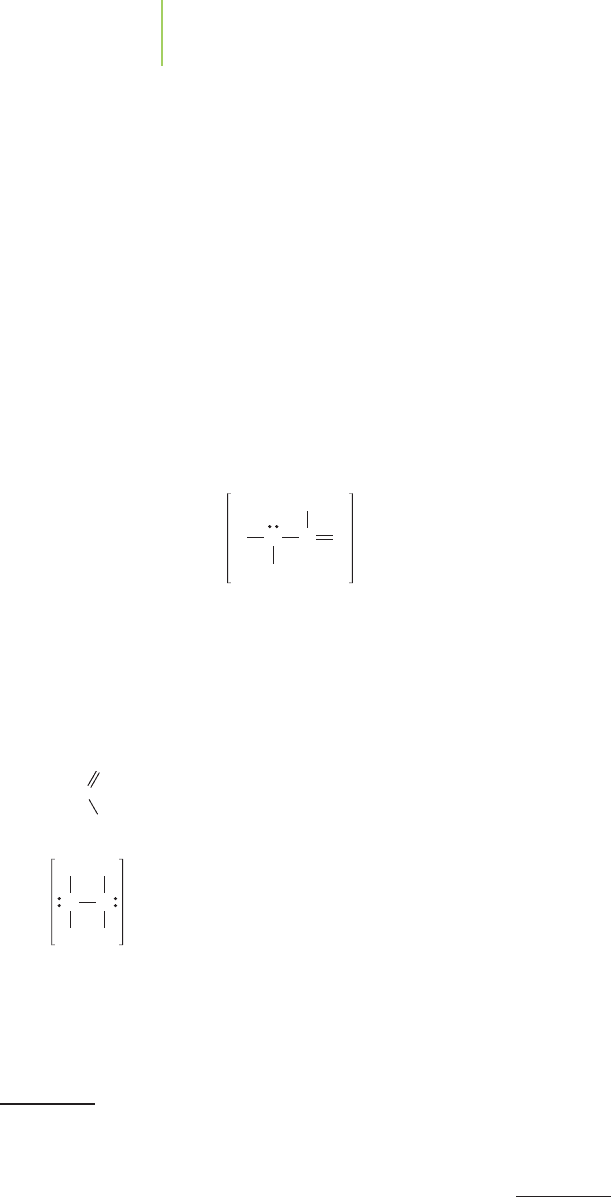
A32 Answers to Practice Exercises and Selected Exercises
91. In the minimum-energy states: a. two; b. none; c. two; d. four
93. a. sp
3
hybridization, tetrahedral shape for orbitals, molecule has
trigonal pyramidal geometry; b. sp
3
d
2
hybridization, octahedral
shape; c. sp
3
hybridization, tetrahedral shape for orbitals, molecule
has bent geometry; d. sp
3
d hybridization, trigonal bipyramid shape
95. NO
2
−
: Nitrogen in both has sp
2
hybridization. Because nitrite has
a lone pair in one of the sp
2
orbitals, the O—N—O bond angle in ni-
trite is reduced from the normal 120° that is found in the nitrate ion.
97. Each carbon in octane will be sp
3
hybridized with tetrahedral
geometry and form a straight chain of carbons. Each C—C bond will
be free to rotate, and the separate molecules can arrange themselves
to closely associate with each other, making the formation of a solid
more likely. 2,2,3,3-Tetramethylbutane has a branched structure.
Each carbon is still sp
3
hybridized, but the center two carbons have
three additional carbons attached to each. The molecule will be like a
large ball, with little possible change in its geometry. This geometry
makes close association between different molecules more difficult
and the process of boiling easier. Octane will not boil as easily as
2,2,3,3-tetramethylbutane.
99. The structure of NH
2
CHO is . The carbon
is sp
2
hybridized (three sigma bonds, no lone pairs) and will be trig-
onal planar. Nitrogen is sp
3
hybridized (three sigma bonds, one lone
pair) and will be tetrahedral. Because the carbon is sp
2
hybridized,
the atoms connected to carbon lie in a single plane, but nitrogen has
tetrahedral geometry, so its two hydrogens are not in the same plane
as the rest of the molecule. The overall molecule is not planar and
will be puckered. 101. a. COC; b. 3.98 × 10
−19
J; c. 239 kJ/mol;
d. blue-green. 103. a. C
18
H
34
O
2
+ H
2
n C
18
H
36
O
2
; b. 3.2 g; c. The
polar end ( ) will interact with the polar water and point down.
104. a.
b. trigonal pyramidal
around each N; c. sp
3
; d. This compound is unlikely to have a color
because it does not have a low-lying orbital to which an electron may
make a transition. The sp
3
hybridization scheme has all four orbitals
used in bond making or holding an electron pair. No empty pi or pi
∗
are present; e. N
2
H
4
+ O
2
n N
2
+ 2H
2
O
H = –590 kJ/mol.
Chapter 10
P10.1 6.7 m/s
2
P10.2 0.979 atm; 744 torr; 0.992 bar; 9.92 ×10
4
Pa
P10.3 1.2 ×10
2
torr P10.4 0.75 mol P10.5 1.3 L P10.6 522 K
P10.7 It is polar and experiences attractive intermolecular forces.
P10.8 0.34 L P10.9 0.036 mol P10.10 0.734 atm P10.11 O
2
:
ideal, 24.4 atm; van der Waals, 23.9 atm; NH
3
: ideal, 24.4 atm,
van der Waals, 21.2 atm P10.12 46.1 g/mol P10.13 53.7 g
P10.14 18.5 kg P10.15 If the masses of two particles are different,
but the particles have the same kinetic energy, their velocities must
be different to offset the difference in mass. As the mass increases,
the velocity must decrease in order for the kinetic energy to remain
the same. P10.16 F
2
P10.17 H
2
effuses 1.4 times as rapidly
as He.
1. These forces are collectively known as van der Waals forces and are
very small because of the large distances between the molecules and
the rapid velocities. 3. High temperature and low pressure
N
H
H
N
H
H
C
OH
O
HN
H
H
C O
5. 8.7 N 7. 8.4 × 10
2
N 9. 1.06 × 10
5
Pa; 106 kPa; 1.05 atm;
797 torr; 1.06 bar; 31.4 in Hg 11. 4.9 lb 13. 3.25 × 10
3
lb
15. 32.2 psi, 2.19 atm 17. 5.5 × 10
2
mm Hg 19. N
2
: 0.656 atm;
O
2
: 0.574 atm 21. 758 mm Hg 23. Ne: 0.306 mm Hg; He: 3.12
mm Hg 25. 9.0 mol, 1.8 mol 27. 5.32 L, 10.1 L 29. 0.847 L, 1.34 L
31. 10.5 L 33. 594 K 35. 0.12 L 37. 0.144 mL
39. a. 0.08205 L·atm·mol
−1
·K
−1
, 8314 L·Pa·mol
−1
·K
−1
; b. 62.36
L·mm·Hg·mol
−1
K
−1
; c. 1.206 L·psi·mol
−1
·K
−1
41. 3.8 × 10
7
L
43. 22.4 L, 51.2 L 45. 0.193 mol 47. 17.1 g/mol 49. 0.755 g/L
51. 2.06 × 10
21
molecules 53. 114 g/mol 55. 1.41 ×10
3
g/mol, No
57. 271 × 10
4
K 59. 8.47 L 61. 12.6 g/L 63. 0.306 atm
65. H
2
is the limiting reagent, 1.33 L 67. 33.9 g/mol 69. F
2
71. 529 L 73. According to the kinetic-molecular theory, the aver-
age kinetic energy of a particle is directly proportional to the tem-
perature. The pressure of a gas inside the cylinder depends on both
the number of collisions with the walls of the container and the
speed of the molecules. As the temperature increases, the speed
increases and both the number and the velocity of the collisions
increase; therefore, the pressure is directly proportional to the
temperature. 75. a. same; b. Cl
2
< ClO
2
; c. ClO
2
; d. ClO
2
77. a. 4.4 × 10
−20
J; b. 1.7 × 10
−20
J; c. 2.0 × 10
−20
J 79. Ar < C
2
H
6
< H
2
81. 411 m/s 83. CO
2
: 376 m/s; H
2
O: 588 m/s 85. Point 1:
The marbles do not have negligible size relative either to the con-
tainer or to the distance between marbles. Point 2: Unless the mar-
bles are dirty and sticky, this should be true because the marbles will
not be strongly attracted to each other. Point 3: If the marbles are
continuously shaken during the demonstration, this should be true.
Point 4: This should also be true during the demonstration if the
marbles are continuously shaken. As the piston is moved, the num-
ber of particle collisions per surface area will change. A smaller vol-
ume will correspond to larger pressures. Point 5: This will be approx-
imately correct. There will be some small energy loss (due to friction
and collisional heating) as the marbles move and collide with each
other and the container. Point 6: This will not be true in the analogy,
but more violent shaking could be applied to show higher tempera-
tures. 87. Xe < SO
3
< CO
2
< N
2
89. 72.7 g/mol 91. Ar 93. Be-
cause the masses are not exactly the same (CO
2
= 44.01 g/mol and
C
3
H
8
= 44.09 g/mol), there will be a slight difference in the effusion
rates. It would be possible, though impractical, to try to separate
these molecules via effusion. 95. 16 cm from the HCl end
97. 0.57% faster 99. a. 1.57 × 10
13
L; b. 1.97 × 10
13
L; c. 1.43 × 10
6
metric tons; d. 1.09 × 10
12
mol 101. 4.75 × 10
24
molecules
103. ideal: 21.8 atm; van der Waals: 22.1 atm 105. ∆T = 894 K;
V
final
= 0.916 V
initial
107. 91.4 g; 338 balloons 109. One of the
considerations is which property is easier to manage: a temperature
of only 20 K or a high pressure of 400 atm. Each requires a different
infrastructure to handle. High pressures require thick walls with
excellent welding, whereas low temperatures require vacuum dewers
(like a thermos), and any variation in temperature causes the hydro-
gen to boil and be lost. 110. a. dispersion only; b. hydrocarbons
behave ideally except at high pressures or low temperatures;
c. 0.0123 mol; d. C
3
H
8
; e. 0.453 L
Chapter 11
P11.1 The size of the London dispersion force between the mole-
cules increases as the size of the molecule increases. Therefore, the
forces between the molecules of dodecane are much larger than the
forces between hexane molecules, which in turn are much larger
than the forces between propane molecules. The larger the force, the
closer the molecules and the stronger they will be held together.
Dodecane is so strongly held that it forms a solid, and hexane is held
strongly enough to cause it to condense to a liquid at room tempera-
ture. P11.2 Propylene glycol has a higher boiling point because the
intermolecular forces will be higher. P11.3 Any molecule with
greater forces will have a higher boiling point and a lower vapor