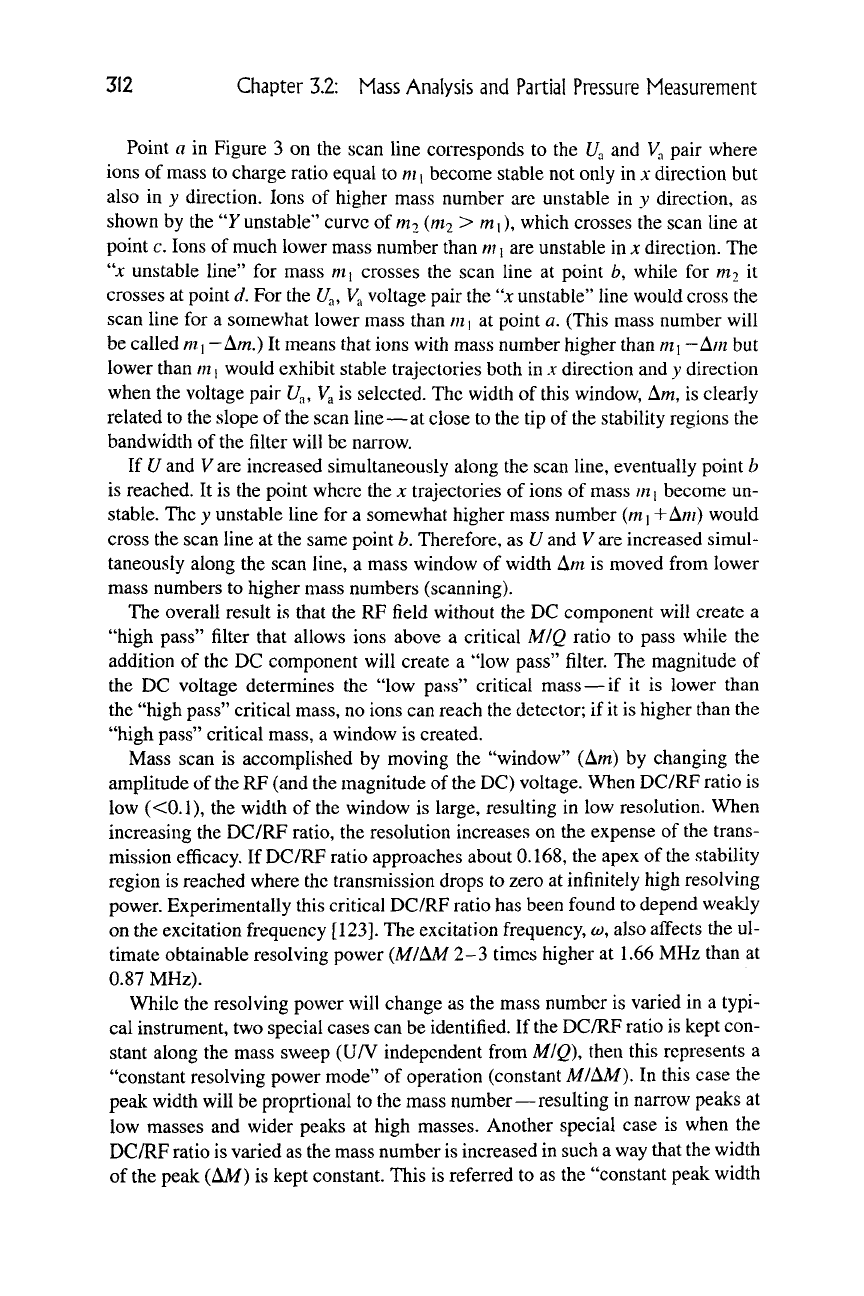
312 Chapter 3.2: Mass Analysis and Partial Pressure Measurement
Point a in Figure 3 on the scan line corresponds to the
U^
and
V^
pair where
ions of mass to charge ratio equal to
m
|
become stable not only in x direction but
also in y direction. Ions of higher mass number are unstable in y direction, as
shown by the "7unstable" curve of
m2
(nii > mi), which crosses the scan line at
point c. Ions of much lower mass number than
m
i
are unstable in x direction. The
"x unstable line" for mass m^ crosses the scan line at point 6, while for m2 it
crosses at point d. For the
U^,
V^
voltage pair the
"JC
unstable" line would cross the
scan line for a somewhat lower mass than nii at point a. (This mass number will
be called
Wi
— Am.) It means that ions with mass number higher than m\ -Am but
lower than
m
i
would exhibit stable trajectories both in x direction and y direction
when the voltage pair
Ur^,
V^
is selected. The width of this window. Am, is clearly
related to the slope of the scan
line
—
at
close to the tip of the stability regions the
bandwidth of the filter will be narrow.
If U and V are increased simultaneously along the scan line, eventually point b
is reached. It is the point where the x trajectories of ions of mass
m
i
become un-
stable. The y unstable line for a somewhat higher mass number (mi -hAw/) would
cross the scan line at the same point b. Therefore, as U and V are increased simul-
taneously along the scan line, a mass window of width Am is moved from lower
mass numbers to higher mass numbers (scanning).
The overall result is that the RF field without the DC component will create a
"high pass" filter that allows ions above a critical M/Q ratio to pass while the
addition of the DC component will create a "low pass" filter. The magnitude of
the DC voltage determines the "low pass" critical mass — if it is lower than
the "high pass" critical mass, no ions can reach the detector; if it is higher than the
"high pass" critical mass, a window is created.
Mass scan is accomplished by moving the "window" (Am) by changing the
amplitude of the RF (and the magnitude of the DC) voltage. When DC/RF ratio is
low (<0.1), the width of the window is large, resulting in low resolution. When
increasing the DC/RF ratio, the resolution increases on the expense of the trans-
mission efficacy. If DC/RF ratio approaches about 0.168, the apex of the stability
region is reached where the transmission drops to zero at infinitely high resolving
power. Experimentally this critical DC/RF ratio has been found to depend weakly
on the excitation frequency
[123].
The excitation frequency,
(o,
also affects the ul-
timate obtainable resolving power (M/iiM 2-3 times higher at 1.66 MHz than at
0.87 MHz).
While the resolving power will change as the mass number is varied in a typi-
cal instrument, two special cases can be identified. If the DC/RF ratio is kept con-
stant along the mass sweep (U/V independent from M/Q), then this represents a
"constant resolving power mode" of operation (constant
MIHsM).
In this case the
peak width will be proprtional to the mass number—resulting in narrow peaks at
low masses and wider peaks at high masses. Another special case is when the
DC/RF ratio is varied as the mass number is increased in such a way that the width
of the peak (AM) is kept constant. This is referred to as the "constant peak width