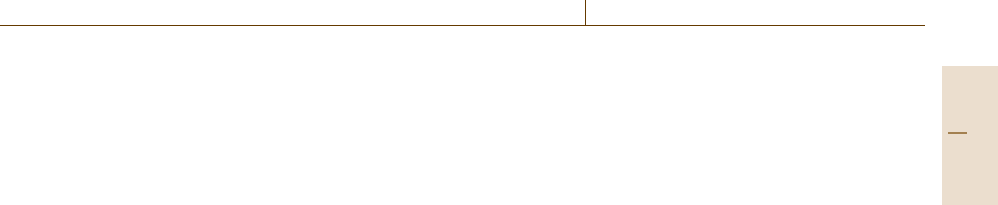
Analytical Chemistry 4.2 Microanalytical Chemical Characterization 179
4.2 Microanalytical Chemical Characterization
Establishing the spatial relationships of the chemical
constituents of materials requires special methods that
build on many of the bulk methods treated in the pre-
ceding portion of this chapter. There may be interest in
locating the placement of a trace chemical constituent
within an engineered structure, or in establishing the
extent of chemical alteration of a part taken out of ser-
vice, or in locating an impurity that is impacting on the
performance of a material. When the question of the rel-
ative spatial locations of different chemical constituents
is at the core of the measurement challenge, methods of
chemical characterization that preserve the structures of
interest during analysis are critical. Not all bulk analyt-
ical methods are suited for surface and/or microanalyt-
ical applications, but many are. In the remainder of this
chapter, some of the more broadly applicable methods
are touched upon, indicating their utility for establishing
chemical composition as a function of spatial location,
in addition to their use for quantitative analysis.
4.2.1 Analytical Electron Microscopy (AEM)
When a transmission electron microscope (TEM)is
equipped with a spectrometer for chemical analysis, it is
usually referred to as an analytical electron microscope.
The two most common chemical analysis techniques
employed by far are energy-dispersive x-ray spectrom-
etry (XEDS) and electron energy-loss spectroscopy
(EELS). In modern TEMs a field emission electron
source is used to generate a nearly monochromatic
beam of electrons. The electrons are then accelerated
to a user-defined energy, typically in the range of
100–400 keV, and focused onto the sample using a se-
ries of magnetic lenses that play an analogous role to
the condenser lens in a compound light microscope. Af-
ter interacting with the sample, the transmitted electrons
are formed into a real image using a magnetic objec-
tive lens. This real image is then further magnified by
a series of magnetic intermediate and projector lenses
and recorded using a charged coupled device (CCD)
camera.
Principles of the Technique. Images with a spatial res-
olution near 0.2 nm are routinely produced using this
technique. In an alternative mode of operation, the con-
denser lenses can be used to focus the electron beam
into a very small spot (less than 1 nm in diameter)
that is rastered over the sample using electrostatic de-
flection coils. By recording the transmitted intensity at
each pixel in the raster, a scanning transmission elec-
tron microscope (STEM) image can be produced. After
a STEM image has been recorded, it can be used to lo-
cate features of interest on the sample and the scan coils
can then be used to reposition the electron beam with
high precision onto each feature for chemical analy-
sis. As the beam electrons are transmitted through the
sample, some of them are scattered inelastically and
produce atomic excitations. Using an EELS spectrom-
eter, a spectrum of the number of inelastic scatters as
a function of energy loss can be produced. Simultane-
ously, an XEDS spectrometer can be used to measure
the energy spectrum of x-rays emitted from the sam-
ple as the atoms de-excite. Both of these spectroscopies
can provide detailed quantitative information about the
chemical structure of the sample with very high spatial
resolution.
In many ways EELS and XEDS are complemen-
tary techniques, and the limitations of one spectroscopy
are often offset by the strengths of the other. Because
elements with low atomic number do not fluoresce effi-
ciently, XEDS begins to have difficulty with elements
lighter than sodium and is difficult or impossible to
use for elements below carbon. In contrast, EELS is
very efficient at detecting light elements. Because EELS
has much better energy resolution than XEDS (≈ 1eV
for EELS and 130 eV for XEDS), it is also capable
of extracting limited information about the bonding
and valence state of the atoms in the analysis region.
The two main drawbacks to EELS are that the sam-
ples need to be very thin compared to XEDS samples,
and that it places greater demands on the analyst, both
experimentally during spectrum acquisition and theo-
retically during interpretation of the results. Because
XEDS works well on relatively thick samples and is eas-
ier to execute, it enjoys widespread use, while EELS is
often considered a more specialized technique.
Nature of the Sample. Perhaps the single most im-
portant drawback to AEM is that all samples must be
thinned to electron transparency. The maximum accept-
able thickness varies with the composition of the sample
and the nature of the analysis sought, but in most cases
the samples must be less than ≈ 500 nm thick. For
quantitative EELS, the samples must be much thinner:
a few tens of nanometers thick at most. Another impor-
tant limitation is that the samples be compatible with
the high vacuum environment required by the electron
optics. Fortunately, a wide array of sample prepara-
Part B 4.2