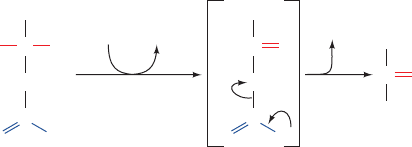
956 Chapter 25. Lipid Metabolism
However, in methylmalonyl-CoA mutase, the AdoCbl is
packed against the N-terminal ends of the barrel’s strands.
2. In free AdoCbl, the Co atom is axially liganded by
an N atom of its DMB group and by the adenosyl residue’s
5¿-CH
2
group (Fig. 25-21). However, in the enzyme, the
DMB has swung aside to bind in a separate pocket and
has been replaced by the side chain of His 610 from the C-
terminal domain. The adenosyl group is not visible in the
structure due to disorder and hence has probably also
swung aside.
3. In nearly all other / barrel–containing enzymes,
the center of the barrel is occluded by large, often branched,
hydrophobic side chains. However, in methylmalonyl-CoA
mutase, the 2-carboxypropyl-CoA’s pantetheine group
binds in a narrow tunnel through the center of the / bar-
rel so as to put the methylmalonyl group of an intact sub-
strate in close proximity to the unliganded face of the
cobalamin ring.This tunnel provides the only direct access
to the active site cavity, thereby protecting the reactive free
radical intermediates that are produced in the catalytic re-
action from side reactions (see below). The tunnel is lined
by small hydrophilic residues (Ser and Thr).
Methylmalonyl-CoA mutase’s substrate binding mode re-
sembles those of several other AdoCbl-containing en-
zymes of known structure, which are collectively unique
among / barrel–containing proteins.
The proposed methylmalonyl-CoA mutase reaction
mechanism (Fig. 25-23) begins with homolytic cleavage of
the cobalamin C¬Co(III) bond (the C and Co atoms each
acquire one of the electrons that formed the cleaved elec-
tron pair bond). The Co ion therefore fluctuates between
its Co(III) and Co(II) oxidation states [the two states are
spectroscopically distinguishable: Co(III) is red and dia-
magnetic (no unpaired electrons), whereas Co(II) is yellow
and paramagnetic (unpaired electrons)]. Note that a ho-
molytic cleavage reaction is unusual in biology; most other
biological bond cleavage reactions occur via heterolytic
cleavage (in which the electron pair forming the cleaved
bond is fully acquired by one of the separating atoms).
The role of AdoCbl in the catalytic process is that of a re-
versible free radical generator. The C¬Co(III) bond is well
suited to this function because it is inherently weak (disso-
ciation energy 109 kJ mol
1
) and appears to be further
weakened through steric interactions with the enzyme. In-
deed, as Fig. 25-22 indicates, the Co atom in methylmalonyl-
CoA mutase has no sixth ligand and hence, as confirmed by
spectroscopic measurements, is in its Co(II) state. The His
N¬Co bond is extremely long (2.5 Å vs 1.9–2.0 Å in vari-
ous other B
12
-containing structures). It is proposed that
this strained and hence weakened bond stabilizes the
Co(II) state with respect to the Co(III) state, thus favoring
the formation of the adenosyl radical and facilitating the
homolytic cleavage through which the catalyzed reaction
occurs (Fig. 23-23). The adenosyl radical presumably ab-
stracts a hydrogen atom from the substrate, thereby facili-
tating the rearrangement reaction through the intermedi-
ate formation of a cyclopropyloxy radical.
d. Succinyl-CoA Cannot Be Directly Consumed
by the Citric Acid Cycle
Methylmalonyl-CoA mutase catalyzes the conversion
of a metabolite to a C
4
citric acid cycle intermediate, not
acetyl-CoA. The route of succinyl-CoA oxidation is there-
fore not as simple as it may first appear. The citric acid
cycle regenerates all of its C
4
intermediates so that these
compounds are really catalysts, not substrates. Conse-
quently, succinyl-CoA cannot undergo net degradation by
citric acid cycle enzymes alone. Rather, in order for a
metabolite to undergo net oxidation by the citric acid cycle,
it must first be converted either to pyruvate or directly to
acetyl-CoA. Net degradation of succinyl-CoA begins with
its conversion, via the citric acid cycle, to malate. At high
concentrations, malate is transported, by a specific trans-
port protein, to the cytosol, where it may be oxidatively de-
carboxylated to pyruvate and CO
2
by malic enzyme
(malate dehydrogenase, decarboxylating):
(We previously encountered this enzyme in the C
4
cycle of
photosynthesis; Fig. 24-41.) Pyruvate is then completely ox-
idized via pyruvate dehydrogenase and the citric acid cycle.
e. Pernicious Anemia Results from
Vitamin B
12
Deficiency
The existence of vitamin B
12
came to light in 1926 when
George Minot and William Murphy discovered that perni-
cious anemia, a rare but often fatal disease of the elderly
characterized by decreased numbers of red blood cells,
low hemoglobin levels (for reasons explained in Section
26-4D), and progressive neurological deterioration (caused
by the accumulation of odd-chain fatty acid residues in
neuronal membranes), can be treated by the daily con-
sumption of large amounts of raw liver (a treatment that
some patients considered worse than the disease). It was
not until 1948, however, after a bacterial assay for antiper-
nicious anemia factor had been developed, that vitamin B
12
was isolated.
Vitamin B
12
is synthesized by neither plants nor animals
but only by a few species of bacteria. Herbivores obtain
their vitamin B
12
from the bacteria that inhabit their gut (in
fact, some animals, such as rabbits, must periodically eat
some of their feces to obtain sufficient amounts of this es-
sential substance). Humans, however, obtain almost all
their vitamin B
12
directly from their diet, particularly from
meat. The vitamin is specifically bound in the intestine by
the glycoprotein intrinsic factor that is secreted by the
stomach.This complex is absorbed by a specific receptor in
the intestinal mucosa, where the complex is dissociated and
the liberated vitamin B
12
transported to the bloodstream.
CO
–
2
C
C
CH
2
O
OO
–
CO
–
2
C
C
CH
2
H
OO
–
CO
2
CH
3
CO
CO
–
2
PyruvateMalate
NADPHNADP
+
H
+
+
HO
JWCL281_c25_940-1018.qxd 4/20/10 1:59 PM Page 956