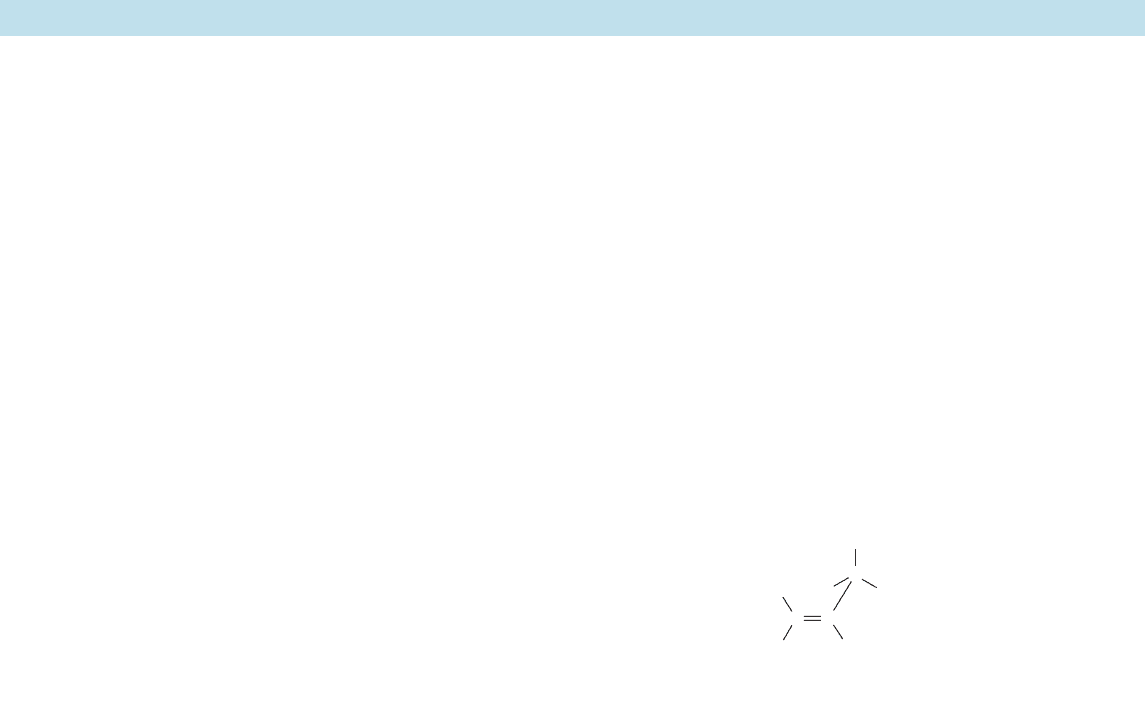
Porter, D.J.T. and Bright, H.J., 3-Carbanionic substrate analogues
bind very tightly to fumarase and aspartase, J. Biol. Chem. 255,
4772–4780 (1980).
Remington, S.J., Structure and mechanism of citrate synthase,
Curr. Top. Cell Regul. 33, 202–229 (1992); and Mechanisms of
citrate synthase and related enzymes (triose phosphate iso-
merase and mandelate racemase), Curr. Opin. Struct. Biol. 2,
730–735 (1992).
Wolodk,W.T., Fraser, M.E., James, M.N.G., and Bridger,W.A.,The
crystal structure of succinyl-CoA synthetase from Escherichia
coli at 2.5 Å resolution, J. Biol. Chem. 269, 10883–10890 (1994).
Zheng, L., Kennedy, M.C., Beinert, H., and Zalkin, H. Mutational
analysis of active site residues in pig heart aconitase, J. Biol.
Chem. 267, 7895–7903 (1992).
Metabolic Poisons
Gibble, G.W., Fluoroacetate toxicity, J. Chem. Educ. 50, 460–462
(1973).
Jones, D.E.H. and Ledingham, K.W.D., Arsenic in Napoleon’s
wallpaper, Nature 299, 626–627 (1982).
Lauble, H., Kennedy, M.C., Emptage, M.H., Beinert,H., and Stout,
C.D., The reaction of fluorocitrate with aconitase and the crys-
tal structure of the enzyme-inhibitor complex, Proc. Natl.
Acad. Sci. 93, 13699–13703 (1996).
Winslow, J.H., Darwin’s Victorian Malady, American Philosophi-
cal Society (1971).
Control Mechanisms
Hurley, J.H., Dean, A.M., Sohl, J.L., Koshland, D.E., Jr., and
Stroud, R.M., Regulation of an enzyme by phosphorylation at
the active site, Science 249, 1012–1016 (1990).
Owen, O.E., Kalhan, S.C., and Hanson, R.W., The key role of
anaplerosis and cataplerosis for citric acid cycle function, J.
Biol. Chem. 277, 30409–30412 (2002).
Reed, L.J., Damuni, Z., and Merryfield, M.L., Regulation of mam-
malian pyruvate and branched-chain ␣-keto-acid dehydroge-
nase complexes by phosphorylation and dephosphorylation,
Curr. Top. Cell. Regul. 27, 41–49 (1985).
Srere, P.A., Sherry, A.D., Malloy, C.R., and Sumegi, B., Chan-
nelling in the Krebs tricarboxylic acid cycle, in Agius, L. and
Sherratt, H.S.A. (Eds.), Channelling in Intermediary Metabo-
lism, pp. 201–217, Portland Press (1997).
Stroud, R.M., Mechanisms of biological control by phosphoryla-
tion, Curr. Opin. Struct. Biol. 1, 826–835 (1991). [Reviews,
among other things, the inactivation of isocitrate dehydroge-
nase by phosphorylation.]
Vélot, C., Mixon, M.B., Teige, M., and Srere, P.A., Model of a
quinary structure between Krebs TCA cycle enzymes: A model
for the metabolon, Biochemistry 36, 14271–14276 (1997).
Vélot, C. and Srere,P.A., Reversible transdominant inhibition of a
metabolic pathway. In vivo evidence of interaction between
two sequential tricarboxylic acid cycle enzymes in yeast. J.
Biol. Chem. 275, 12926–12933 (2000).
Problems 821
1. Trace the course of the radioactive label in [2-
14
C]glucose
through glycolysis and the citric acid cycle.At what point(s) in the
cycle will the radioactivity be released as
14
CO
2
? How many turns
of the cycle will be required for complete conversion of the ra-
dioactivity to CO
2
? Repeat this problem for pyruvate that is
14
C-
labeled at its methyl group.
2. The reaction of glutathione reductase with an excess of
NADPH in the presence of arsenite yields a nonphysiological
four-electron reduced form of the enzyme. What is the chemical
nature of this catalytically inactive species?
3. Two-electron reduced dihydrolipoyl dehydrogenase (EH
2
),
but not the oxidized enzyme (E), reacts with iodoacetate
(ICH
2
COO
⫺
) to yield an inactive enzyme. Explain.
4. Given the following information, calculate the physiologi-
cal ⌬G of the isocitrate dehydrogenase reaction at 25°C and pH
7.0: [NAD
⫹
]/[NADH] ⫽ 8; [␣-ketoglutarate] ⫽ 0.1 mM; [isoci-
trate] ⫽ 0.02 mM; assume standard conditions for CO
2
(⌬G°¿ is
given in Table 21-2). Is this reaction a likely site for metabolic con-
trol? Explain.
5. The oxidation of acetyl-CoA to two molecules of CO
2
in-
volves the transfer of four electron pairs to redox coenzymes. In
which of the cycle’s reactions do these electron transfers occur?
Identify the redox coenzyme in each case. For each reaction, draw
the structural formulas of the reactants, intermediates, and
products and show, using curved arrows, how the electrons are
transferred.
6. The citrate synthase reaction has been proposed to proceed
via the formation of the enol(ate) form of acetyl-CoA. How, then,
would you account for the observation that
3
H is not incorporated
into acetyl-CoA when acetyl-CoA is incubated with citrate syn-
thase in
3
H
2
O?
7. Malonate is a competitive inhibitor of succinate in the suc-
cinate dehydrogenase reaction. (a) Sketch the graphs that would
be obtained on plotting 1/v versus 1/[succinate] at three different
malonate concentrations. Label the lines for low, medium, and
high [malonate]. (b) Explain why increasing the oxaloacetate con-
centration in a cell can overcome malonate inhibition.
8. Krebs found that malonate inhibition of the citric acid cycle
could be overcome by raising the oxaloacetate concentration. Ex-
plain the mechanism of this process in light of your findings in
Problem 7.
*9. (2R,3R)-2-Fluorocitrate contains F in the pro-S carboxy-
methyl arm of citrate [note that the rules of organic nomenclature re-
quire that atom C2 in citrate (Fig. 21-20) be renumbered as C4 in
(2R,3R)-2-fluorocitrate]. This compound, but not its diastereomer, is
a potent inhibitor of aconitase. (a) Draw the aconitase-catalyzed re-
action pathway of (2R,3R)-2-fluorocitrate assuming it follows the
same reaction pathway as citrate (Fig. 21-20). (b) Aconitase, in fact,
does not catalyze the foregoing reaction with (2R,3R)-2-fluorocitrate
but, rather, yields the following tight-binding inhibitor:
Draw an alternative aconitase-catalyzed reaction that would gener-
ate this inhibitor. (c) Draw the aconitase-catalyzed reaction of
H
–
OOC
H
OH
COO
–
COO
–
C
C
C
PROBLEMS
JWCL281_c21_789-822.qxd 3/17/10 11:34 AM Page 821