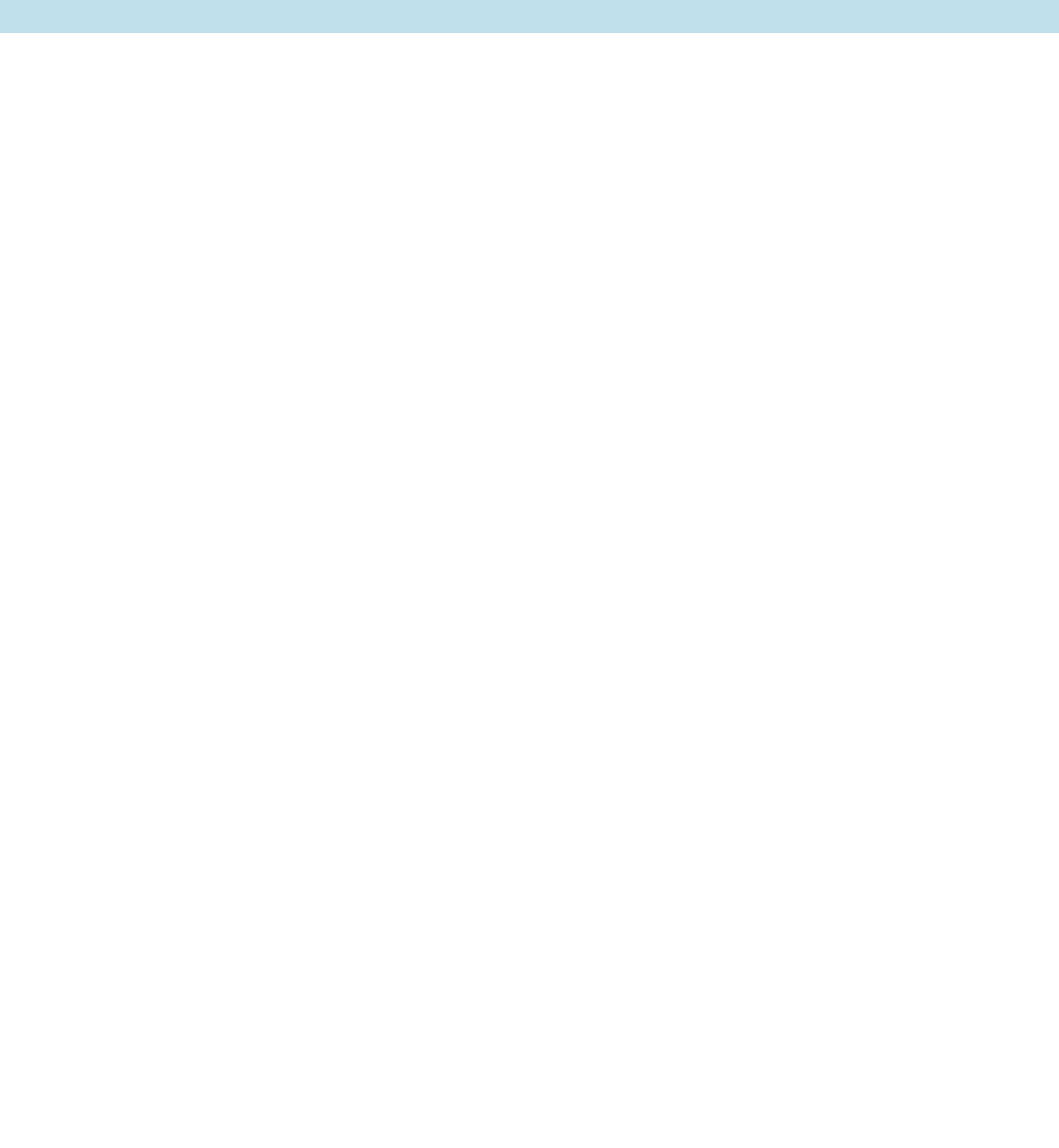
820 Chapter 21. Citric Acid Cycle
History
Holmes, F.L., Hans Krebs: Vol. 1: The Formation of a Scientific
Life, 1900–1933; and Vol. 2: Architect of Intermediary Metabo-
lism, 1933–1937, Oxford University Press (1991 and 1993).
[The biography of the discoverer of the citric acid cycle
through the time of its discovery.]
Kornberg, H.L., Tricarboxylic acid cycles, BioEssays 7, 236–238
(1987). [A historical synopsis of the intellectual background
leading to the discovery of the citric acid cycle.]
Krebs, H.A., The history of the tricarboxylic acid cycle, Perspect.
Biol. Med. 14, 154–170 (1970).
Pyruvate Dehydrogenase Multienzyme Complex
Frank, R.A.W., Pratap, J.V., Pei, X.Y., Perham, R.N., and Luisi,
B.F., The molecular origins of specificity in the assembly of a
multienzyme complex, Structure 13, 1119–1130 (2005). [The X-
ray structure of B. stearothermophilus E1 ⴢ TPP ⴢ PSBD.]
Frank, R.A.W.,Titman, C.M., Pratap, J.V., Luisi, B.F., and Perham,
R.N., A molecular switch and proton wire synchronize the ac-
tive sites in thiamine enzymes, Science 306, 872–876 (2004).
Izard,T., Ævarsson,A.,Allen, M.D.,Westphal,A.H., Perham, R.N.,
de Kok, A., and Hol, W.G.J., Principles of quasi-equivalence
and euclidean geometry govern the assembly of cubic and do-
decahedral cores of pyruvate dehydrogenase, Proc. Natl.Acad.
Sci. 96, 1240–1245 (1999).
Karplus, P.A.and Schulz, G.E., Refined structure of glutathione re-
ductase at 1.54 Å resolution, J. Mol. Biol. 195, 701–729 (1987).
Karplus,P.A.and Schulz,G.E., Substrate binding and catalysis by glu-
tathione reductase derived from refined enzyme:substrate crystal
structures at 2Å resolution, J. Mol. Biol. 210, 163–180 (1989).
Lengyel, J.S., Stott, K.M., Wu, X., Brooks, B.R., Balbo,A., Schuck,
P., Perham, R.N., Subramaniam, S., and Milne, J.L.S., Extended
polypeptide linkers establish the spatial architecture of a pyru-
vate dehydrogenase multienzyme complex, Structure 16,
93–103 (2008).
Mande, S.S., Sarfaty, S.,Allen, M.D., Perham, R.N., and Hol,W.G.J.,
Protein–protein interactions in the pyruvate dehydrogenase
complex: dihydrolipoamide dehydrogenase complexed with
the binding domain of dihydrolipoamide acetyltransferase,
Structure 4, 277–286 (1996).
Mattevi, A., Obmolova, G., Sokatch, J.R., Betzel, C., and Hol,
W.G.J., The refined crystal structure of Pseudomonas putida
lipoamide dehydrogenase complexed with NAD
⫹
at 2.45 Å
resolution, Proteins 13, 336–351 (1992); and Mattevi, A.,
Schierbeek, A.J., and Hol, W.G.J., Refined crystal structure of
lipoamide dehydrogenase from Azotobacter vinelandii at 2.2 Å
resolution. A comparison with the structure of glutathione re-
ductase, J. Mol. Biol. 220, 975–994 (1991).
Milne, J.L.S.,Wu, X., Borgnia, M.J., Lengyel, J.S., Brooks, B.R., Shi,
D., Perham, R.N., and Subramaniam, S., Molecular structure of
a 9-MDa icosahedral pyruvate dehydrogenase subcomplex
containing the E2 and E3 enzymes using cryoelectron mi-
croscopy, J. Biol. Chem. 281, 4364–4370 (2006).
Patel, M.S. and Korotchkina, L.G., The biochemistry of the pyru-
vate dehydrogenase complex, Biochem. Mol. Biol. Educ. 31,
5–15 (2003).
Perham, R.N., Swinging arms and swinging domains in multifunc-
tional enzymes: Catalytic machines for multistep reactions,
Annu. Rev. Biochem. 69, 961–1004 (2000). [An authoritative
review on multienzyme complexes.]
Reed, L.J.,A trail of research from lipoic acid to ␣-keto acid dehy-
drogenase complexes, J. Biol. Chem. 276, 38329–38336 (2001).
[A scientific memoir.]
Roche, T.E., Baker, J.C.,Yan, X., Hiromasa, Y., Gong, X., Peng, T.,
Dong,J.,Turkan,A., and Kasten, S.E., Distinct regulatory prop-
erties of pyruvate dehydrogenase kinase and phosphatase iso-
forms, Prog. Nucleic Acid Res. Mol. Biol. 70, 33–75 (2001).
Williams, C.H., Jr., Lipoamide dehydrogenase, glutathione reduc-
tase, thioredoxin reductase, and mercuric ion reductase—A
family of flavoenzyme transhydrogenases, in Müller, F. (Ed.),
Chemistry and Biochemistry of Flavoenzymes, Vol. III,
pp. 121–211, CRC Press (2000).
Zhou, Z.H., McCarthy, D.B., O’Connor, C.M., Reed, L.J., and
Stoops, J.K., The remarkable structural and functional organi-
zation of the eukaryotic pyruvate dehydrogenase complexes,
Proc. Natl. Acad. Sci. 98, 14802–14807 (2001).
Enzymes of the Citric Acid Cycle
Beinert, H., Kennedy, M.C., and Stout, D.C., Aconitase as iron-
sulfur protein, enzyme, and iron-regulatory protein, Chem.
Rev. 96, 2335–2374 (1996).
Bolduc, J.M., Dyer, D.H., Scott, W.G., Singer, P., Sweet, R.M.,
Koshland, D.E., Jr., and Stoddard, B.L., Mutagenesis and Laue
structures of enzyme intermediates: Isocitrate dehydrogenase,
Science 268, 1312–1318 (1995).
Cleland, W.W. and Kreevoy, M.M., Low-barrier hydrogen bonds
and enzymic catalysis, Science 264, 1887–1890 (1994).
Frey, P.A. and Hegeman, A.D., Enzymatic Reaction Mechanisms,
Oxford (2007). [Contains discussions of the mechanisms of
various citric acid cycle enzymes.]
Huynen, M.A., Dandekar,T., and Bork, P.,Variation and evolution
of the citric-acid cycle: a genomic perspective, Trends Micro-
biol. 7, 281–291 (1999). [Discusses how genomic studies can al-
low reconstruction of metabolic pathways, even when some
enzymes appear to be missing.]
Karpusas, M., Branchaud, B., and Remington, S.J., Proposed
mechanism for the condensation reaction of citrate synthase:
1.9-Å structure of the ternary complex with oxaloacetate and
carboxymethyl coenzyme A, Biochemistry 29, 2213–2219
(1990).
Kurz, L.C., Nakra, T., Stein, R., Plungkhen, W., Riley, M., Hsu, F.,
and Drysdale, G.R., Effects of changes in three catalytic
residues on the relative stabilities of some of the intermediates
and transition states in the citrate synthase reaction, Biochem-
istry 37, 9724–9737 (1998).
Lauble, H., Kennedy, M.C., Beinert, H., and Stout, D.C., Crystal
structures of aconitase with isocitrate and nitroisocitrate
bound, Biochemistry 31, 2735–2748 (1992).
Mulholland, A.J., Lyne, P.D., and Karplus, M., Ab initio QM/MM
study of the citrate synthase mechanism. A low-barrier hydro-
gen bond is not involved, J. Am. Chem. Soc. 122, 534–535
(2000).
REFERENCES
5 The Amphibolic Nature of the Citric Acid Cycle Sev-
eral anabolic pathways cataplerotically utilize citric acid cycle
intermediates as starting materials. These essential substances
are replaced by anaplerotic reactions of which the major one
is synthesis of oxaloacetate from pyruvate and CO
2
by pyru-
vate carboxylase.
JWCL281_c21_789-822.qxd 6/5/10 9:53 AM Page 820