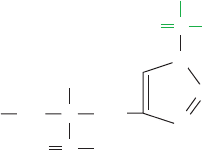
involved. Four phosphorylated protein intermediates have
been identified, indicative of five phosphoryl transfers:
The transport process occurs as follows (Fig. 20-24):
1. PEP phosphorylates EI at N3 (N
ε
) of His 189 to form
a reactive phosphohistidine adduct.
2. The phosphoryl group is transferred to N1 (N
␦
) of
His 15 on HPr. His is apparently a favored phosphoryl
group acceptor in phosphoryl-transfer reactions. It also
participates in the phosphoglycerate mutase reaction of
glycolysis (Section 17-2Ha).
3. HPr
⬃
P continues the phosphoryl-transfer chain by
phosphorylating EIIA
glc
at N3 of His 90.
4. The fourth phosphoryl transfer is to Cys 421 of EIIB
glc
.
5. The phosphoryl group is finally transferred from
EIIB
glc
to glucose, which, in the process, is transported
across the membrane by EIIC
glc
. Glucose is released into
the cytoplasm only after it has been phosphorylated to
glucose-6-phosphate (G6P).
Thus the transport of glucose is driven by its indirect, exergonic
phosphorylation by PEP. The PTS is an energy-efficient sys-
tem since only one ATP equivalent is required to both trans-
port and phosphorylate glucose. When the active transport
and phosphorylation steps occur separately, as they do in
many cells, two ATPs are hydrolyzed per glucose processed.
a. Bacterial Sugar Transport Is
Genetically Regulated
The PTS is more complex than the other transport sys-
tems we have encountered, probably because it is part of a
complicated regulatory system governing sugar transport.
When any of the sugars transported by the PTS is abun-
dant, the active transport of sugars, which enter the cell via
other transport systems, is inhibited.This inhibition, called
catabolite repression, is mediated through the cAMP con-
centration (Section 31-3C). cAMP activates the transcrip-
tion of genes that encode various sugar transport proteins,
including lactose permease (Section 20-4B). The presence
of glucose results in a decrease in [cAMP], which, in turn,
represses the synthesis of these other sugar transport pro-
teins. Direct inhibition of the sugar transport proteins
themselves, as well as of certain enzymes, also occurs.
The mechanism for control of [cAMP] is thought to reside
in EIIA
glc
,which is transiently phosphorylated in Step 3 of the
PTS transport process (Fig.20-24).When glucose is plentiful,
this enzyme is present mostly in its dephospho form since
CH
2
NH
Phosphohistidine residue
PO
O
–
O
–
C
C
H
3
5
4
O
N
N
CH
2
1
PEP S EI S HPr S EIIA
glc
S EIIBC
glc
S glucose
EIIA
glc
⬃
P rapidly transfers its phosphoryl group through
EIIBC
glc
to glucose. Under these conditions, adenylate cy-
clase is inactive, although whether dephospho EIIA
glc
in-
hibits this enzyme or EIIA
glc
⬃
P activates it is unclear.
However, dephospho EIIA
glc
binds to and inhibits many
non-PTS transporters and enzymes that participate in the
metabolism of sugars other than glucose (the metabolite of
choice for many bacteria), including lactose permease and
glycerol kinase (Section 17-5A). In the absence of glucose,
EIIA
glc
is converted to EIIA
glc
⬃
P, thereby relieving the inhi-
bition of non-PTS transporters. In addition, adenylate cy-
clase is activated to produce cAMP, which, in turn, induces
the increased production of some of the non-PTS trans-
porters and enzymes that EIIA
glc
inhibits. This is a form of
energy conservation for the cell.Why synthesize the proteins
required for the transport and metabolism of all sugars
when the metabolism of only one sugar at a time will do?
b. The X-Ray Structure of EIIA
glc
in
Complex with Glycerol Kinase
The X-ray structures of EIIA
glc
, both alone and in com-
plex with one of its regulatory targets, glycerol kinase,
which were determined by James Remington and Rose-
man, have revealed how EIIA
glc
inhibits at least some of its
targets and why EIIA
glc
⬃
P does not do so. EIIA
glc
con-
tains two His residues, His 75 and His 90, that are required
for phosphoryl transfer, although only His 90 is necessary
for EIIA
glc
to accept a phosphate from HPr. The X-ray
structure of E. coli EIIA
glc
alone reveals that these two His
residues lie in close proximity (their N3 atoms are 3.3 Å
apart) in a depression on the surface of the protein that is
surrounded by a remarkable ⬃18-Å-diameter hydropho-
bic ring consisting of 11 Phe, Val, and Ile side chains.
The X-ray structure of EIIA
glc
in complex with glycerol
kinase (Fig. 20-25) confirms that this hydrophobic gasket is
indeed the site of interaction between the two proteins and
reveals how the phosphorylation of His 90 disrupts this inter-
action.The two active site His residues, which are completely
buried within the hydrophobic interaction surface, coordi-
nate a previously unanticipated Zn
2⫹
ion, which is addition-
ally coordinated to Glu 478 of glycerol kinase and a water
molecule. The phosphorylation of EIIA
glc
His 90 to yield EI-
IA
glc
⬃
P no doubt disrupts this intermolecular interaction,
thereby releasing glycerol kinase and reversing its inhibition.
E. ABC Transporters
The ABC transporters, which widely occur in all kingdoms of
life, are named for their highly conserved, ⬃100-residue ATP-
binding cassette. They form a large superfamily of transmem-
brane proteins that collectively transport a wide variety of sub-
stances across membranes, including ions, sugars, peptides,
lipids, metabolites, and numerous toxins and drugs. For exam-
ple, the acquired resistance of cancer cells to chemotherapeu-
tic agents is often due to the selection of cells that overexpress
the ABC transporter named multidrug resistance (MDR)
transporter (also called P-glycoprotein), which pumps a wide
assortment of amphiphilic substances—including many
drugs—out of the cell. Similar proteins in bacteria are, in
many cases, responsible for their antibiotic resistance. The
766 Chapter 20. Transport Through Membranes
JWCL281_c20_744-788.qxd 6/4/10 12:16 PM Page 766