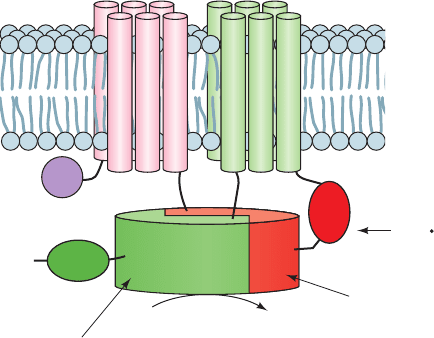
greatly increases the usual ⬃500-D size limit for an effec-
tive drug candidate (Section 15-4Ba). Moreover,a large lig-
and is unlikely to enter the bloodstream and hence would
have minimal side effects. Consequently, the synthesis of
multivalent ligands that simultaneously bind with high
affinity to all five receptor binding sites on an AB
5
mole-
cule has yielded promising lead compounds against CT
and LT.
e. Pertussis Toxin ADP-Ribosylates G
i
Bordetella pertussis, the bacterium that causes pertussis
(whooping cough; a disease that is still responsible for
⬃300,000 infant deaths per year worldwide), produces an
AB
5
protein, pertussis toxin (PT), that ADP-ribosylates a
specific Cys residue in G
i
. In doing so, it prevents G
i
from
exchanging its bound GDP for GTP and therefore from in-
hibiting AC. PT’s X-ray structure, determined by Randy
Read, reveals that its A and B subunits are structurally ho-
mologous to those of CT and LT, although the A subunit of
PT extends from the opposite face of its B pentamer rela-
tive to that in CT. Moreover, PT’s B pentamer consists of
four different subunits (one in two copies), each of which is
only ⬃15% identical to the B subunits of CT and LT.
f. Receptors Are Subject to Desensitization
One of the hallmarks of biological signaling systems is
that they adapt to long-term stimuli by reducing their re-
sponse to them, a process named desensitization. These sig-
naling systems therefore respond to changes in stimulation
levels rather than to their absolute values. What is the mech-
anism of desensitization? In the case of -adrenergic re-
ceptors, an epinephrine–receptor complex, but not recep-
tor alone, is phosphorylated at one or more of the Ser or
Thr residues on its C-terminal tail by -adrenergic recep-
tor kinase (ARK). This cytosolic protein is recruited to
the plasma membrane through its interaction with G
when it is not bound to G
s
, which is also a consequence of
receptor activation. The phosphorylation of the receptor
decreases the ability of epinephrine to influence it, at least
in part by reducing the receptor’s epinephrine-binding
affinity. The phosphorylated receptor, in turn, is bound by
either of two 78% identical proteins known as -arrestins
in a way that blocks the activated receptor from activating
its target G protein. Moreover, the -arrestin binds to the
adaptor protein AP2 in clathrin-coated pits (Section 12-4Cd),
whereupon the -adrenergic receptor–-arrestin complex
is endocytotically sequestered (Section 12-5Bc) in spe-
cialized vesicles. The receptor’s otherwise extracellular,
epinephrine-binding surface then faces the interior of the
vesicle and the -arrestin is located on its outside, from
which it is subsequently released. The vesicles are devoid
of both heterotrimeric G protein and AC,thus further attenu-
ating the cell’s response to epinephrine. If, however, the ep-
inephrine level is reduced, the receptor is slowly dephos-
phorylated by a phosphatase and exocytotically returned
to the cell surface, thus restoring the cell’s epinephrine sen-
sitivity.Alternatively, the vesicles may fuse with endosomes
for the delivery of their contents to lysosomes, where the
receptor is proteolytically degraded (Fig. 12-91). In this latter
case, the restoration of epinephrine sensitivity requires the
synthesis of additional receptor.
ARK is a member of a family of seven proteins known
as GCPR kinases (GRKs) that,together with several other
types of protein kinases including PKA, phosphorylate the
C-terminal tails and/or the cytosolic loops of most agonist-
occupied GPCRs. As above, the phosphorylated GPCRs
are bound by a -arrestin and sequestered into endocytotic
vesicles, thereby isolating them from their corresponding
heterotrimeric G proteins. Moreover, the sites of a given
GCPR that are phosphorylated vary in a tissue-specific
way that provides further regulatory flexibility.
D. Adenylate Cyclases
The heterotrimeric G proteins G
s
and G
i
function to con-
trol the activities of adenylate cyclase (AC). In fact, mam-
mals have 9 known membrane-bound isoforms of AC,AC1
through AC9 (alternatively, AC-I through AC-IX), which
are each expressed in a tissue-specific manner and differ
in their regulatory properties. These ⬃120-kD transmem-
brane glycoproteins each consist of a small N-terminal do-
main (N), followed by two repeats of a unit consisting of a
transmembrane domain (M) followed by two consecutive
cytoplasmic domains (C), thus forming the sequence
NM
1
C
1a
C
1b
M
2
C
2a
C
2b
(Fig. 19-23). The ⬃30% identical C
1a
and C
2a
domains associate to form the AC’s catalytic core,
whereas C
1b
, as well as C
1a
and C
2a
, bind regulatory mole-
cules. Thus, G
i
inhibits AC1, 5, and 6 by binding to C
1a
;G
s
activates all AC isoforms but AC9 by binding to C
2a
;G
in-
hibits AC1, 3, and 8 but activates AC2, 4, and 7 by binding
Section 19-2. Heterotrimeric G Proteins 697
Figure 19-23 Schematic diagram of a typical mammalian AC.
The M
1
and M
2
domains are each predicted to contain six
transmembrane helices. C
1a
and C
2a
form the enzyme’s
pseudosymmetric catalytic core. The domains with which various
regulatory proteins are known to interact are indicated. [After
Tesmer, J.J.G. and Sprang, S.R., Curr. Opin. Struct. Biol. 8, 713
(1998).]
N
C
C
1a
C
2a
G
iα
G
sα,
PKC, G
βγ
M
1
M
2
ATP
2Mg
2+
cAMP + PP
i
C
2b
C
1b
Ca
2+
,
Ca
2+
CaM,
PKA
JWCL281_c19_671-743.qxd 3/16/10 7:16 PM Page 697