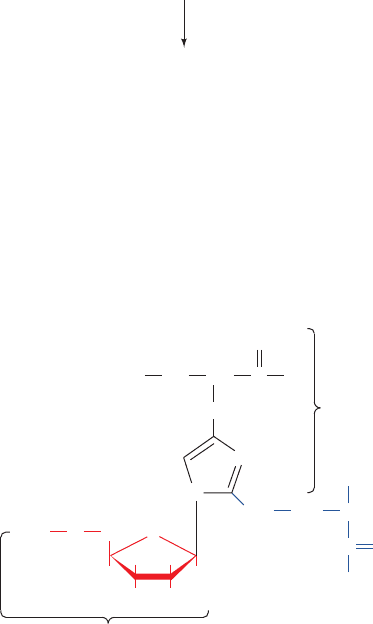
The pathogenic effects of diphtheria are prevented, as
was discovered in the 1880s, by immunization with toxoid
(formaldehyde-inactivated toxin). Individuals who have
contracted diphtheria are treated with antitoxin from
horse serum, which binds to and thereby inactivates DT, as
well as with antibiotics to combat the bacterial infection.
DT is a member of the family of bacterial toxins that in-
cludes cholera toxin (CT) and pertussis toxin (PT; Section
19-2Ce). It is a monomeric 535-residue protein that is readily
cleaved past its Arg residues 190, 192, and 193 by trypsin
and trypsinlike enzymes. This hydrolysis occurs around the
time diphtheria toxin encounters its target cell, yielding
two fragments, A and B, which, nevertheless, remain linked
by a disulfide bond. The B fragment’s C-terminal domain
binds to a specific receptor on the plasma membrane of
susceptible cells, thereby inducing DT’s uptake into the en-
dosome (Fig. 12-91) via receptor-mediated endocytosis
(Section 12-5Bc; free fragment A is devoid of toxic activ-
ity).The endosome’s low pH of 5 triggers a conformational
change in the B fragment’s N-terminal domain, which then
inserts into the endosomal membrane so as to facilitate the
entry of the A fragment into the cytoplasm. The disulfide
bond linking the A and B subunits is then cleaved by the
cytoplasm’s reducing environment.
Within the cytosol, the A fragment catalyzes the ADP-
ribosylation of eEF2 by NAD
,
thereby inactivating this elongation factor. Since the A
fragment acts catalytically, one molecule is sufficient to
ADP-ribosylate all of a cell’s eEF2s, which halts protein
synthesis and kills the cell. Only a few micrograms of diph-
theria toxin are therefore sufficient to kill an unimmu-
nized individual.
Diphtheria toxin specifically ADP-ribosylates a modi-
fied His residue on eEF2 known as diphthamide:
CH
2
CH
2
CH
2
NH CH
O
HH
H
H
N
N
OH OH
ADP-Ribosylated diphthamide
OADP
ADP-ribosyl group
His residue
CH
2
NH
2
CH
CO
C
O
N(CH
3
)
3
+
diphtheria toxin
(inactive)
(active)
+ NAD
+
eEF2
DP-ribosyl-eEF2 + Nicotinamide + H
+
Diphthamide occurs only in eEF2 (not even in its bacter-
ial counterpart, EF-G), which accounts for the specificity
of diphtheria toxin in exclusively modifying eEF2 (recall
that CT ADP-ribosylates a specific Arg residue on G
s
and PT ADP-ribosylates a specific Cys residue on G
ia
;
Section 19-2C). Since diphthamide occurs in all eukary-
otic eEF2s, it probably is essential to eEF2 activity. Yet,
certain mutant cultured animal cells, which have unim-
paired capacity to synthesize proteins, lack the enzymes
that post-translationally modify His to diphthamide
(although mutating the diphthamide His to Asp, Lys, or
Arg inactivates translation). Perhaps the diphthamide
residue has a control function.
4 CONTROL OF EUKARYOTIC
TRANSLATION
The rates of ribosomal initiation on prokaryotic mRNAs
differ by factors of up to 100, a variation that is largely a
consequence of their different Shine–Dalgarno se-
quences. Moreover, the genes forming an operon are of-
ten expressed in decreasing molar amounts from the
operon’s 5¿ end to its 3¿ end. For example, the proteins
specified by the E. coli lac operon (Section 31-1Ab), -
galactosidase, galactose permease, and thiogalactoside
transacetylase, are produced in molar ratios of 10:5:2.
Such polarity may arise when the initiation codon of a
gene that lacks a Shine–Dalgarno sequence is very near
the Stop codon of its upstream gene, a situation that oc-
curs most often when the Stop codon overlaps the initia-
tion codon as in the sequence AUGA. The translation of
the upstream gene will then be required for the transla-
tion of the downstream gene, a phenomenon termed
translational coupling. The polarity arises because a ribo-
some often dissociates from the mRNA on encountering
the upstream gene’s Stop codon.Alternatively, an mRNA
may fold in a way that masks an internal Shine–Dalgarno
sequence, for example, by the base pairing of a segment
adjacent to the Shine–Dalgarno sequence to a down-
stream element of the preceding gene. Such Shine–Dal-
garno sequences only become available when a ribosome
that is translating the preceding gene disrupts the folded
structure.
Genetic expression in prokaryotes is largely transcrip-
tionally controlled (Section 31-3). This is apparently be-
cause prokaryotic mRNAs have lifetimes of only a few
minutes and, hence, it is a more efficient use of resources to
control their transcription. Nevertheless, the expression of
certain prokaryotic genes is translationally controlled,
most notably those encoding the ribosomal proteins
(which comprise 10% of cellular proteins), which must be
produced in equimolar amounts. The production of riboso-
mal proteins is controlled, in part, through a process in
which a ribosomal protein binds to the mRNA of the
operon encoding it in the vicinity of a translational start
site located near the mRNA’s 5¿ end so as to inhibit its
translational initiation. However, each such protein binds
1398 Chapter 32. Translation
JWCL281_c32_1338-1428.qxd 9/7/10 2:23 PM Page 1398