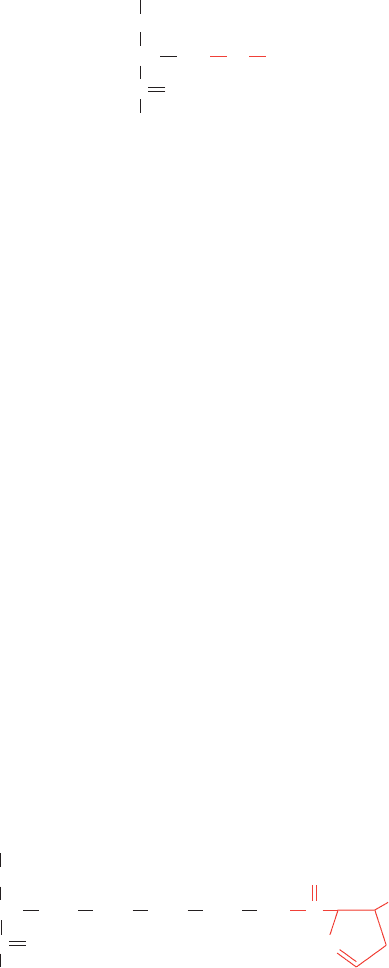
No prokaryotic or eukaryotic cytoplasmic tRNA is
known to participate in a nonwobble pairing combination.
There is, however, no known instance of such a tRNA with
an A in its third anticodon position, which suggests that the
consequent A U pair is not permitted.The structural basis
of wobble pairing is poorly understood, although it is clear
that it is influenced by base modifications.
A consideration of the various wobble pairings indi-
cates that at least 31 tRNAs are required to translate all 61
coding triplets of the genetic code (there are 32 tRNAs in
the minimal set because translational initiation requires a
separate tRNA; Section 32-3Ca). Most cells have 32
tRNAs, some of which have identical anticodons. In fact,
mammalian cells have 150 tRNAs. Nevertheless, all
isoaccepting tRNAs in a cell are recognized by a single
aminoacyl–tRNA synthetase.
c. Some Mitochondrial tRNAs Have More Permissive
Wobble Pairings than Other tRNAs
The codon recognition properties of mitochondrial
tRNAs must reflect the fact that mitochondrial genetic codes
are variants of the “standard” genetic code (Table 32-3). For
instance, the human mitochondrial genome, which consists
of only 16,569 bp, encodes 22 tRNAs (together with 2 ribo-
somal RNAs and 13 proteins). Fourteen of these tRNAs each
read one of the synonymous pairs of codons indicated in
Tables 32-2 and 32-3 (MNX, where X is either C or U or else
A or G) according to normal G U wobble rules:The tRNAs
have either a G or a modified U in their third anticodon po-
sition that, respectively, permits them to pair with codons
having X C or U or else X A or G. The remaining 8
tRNAs,which,contrary to wobble rules,each recognize one of
the groups of four synonymous codons (MNY, where Y A,
C, G, or U), all have anticodons with a U in their third posi-
tion. Either this U can somehow pair with any of the four
bases or these tRNAs read only the first two codon positions
and ignore the third.Thus, not surprisingly, many mitochon-
drial tRNAs have unusual structures in which, for example,
the GTCRA sequence (Fig. 32-9) is missing,or, in the most
bizarre case, a tRNA
Ser
lacks the entire D arm.
d. Frequently Used Codons Are Complementary to
the Most Abundant tRNA Species
The analysis of the base sequences of several highly ex-
pressed structural genes of S. cerevisiae has revealed a re-
markable bias in their codon usage. Only 25 of the 61 cod-
ing triplets are commonly used. The preferred codons are
those that are most nearly complementary, in the
Watson–Crick sense, to the anticodons in the most abundant
species in each set of isoaccepting tRNAs. Furthermore,
codons that bind anticodons with two consecutive G C
pairs or three A U pairs are avoided so that the preferred
codon–anticodon complexes all have approximately the
same binding free energies. A similar phenomenon occurs
in E. coli, although several of its 22 preferred codons differ
from those in yeast. The degree with which the preferred
codons occur in a given gene is strongly correlated, in both
organisms, with the gene’s level of expression (the meas-
ured rates of aminoacyl–tRNA selection in E. coli span a
25-fold range). This, it has been proposed, permits the
mRNAs of proteins that are required in high abundance to
be rapidly and smoothly translated.
e. Selenocysteine and Pyrrolysine Are Carried by
Specific tRNAs
Although it is widely stated, even in this text, that pro-
teins are synthesized from the 20 “standard” amino acids,
that is, those specified by the “standard” genetic code, some
organisms, as Theresa Stadtman discovered, use a twenty-
first amino acid, selenocysteine (Sec; alternatively SeCys),
in synthesizing a few of their proteins:
Selenium, a biologically essential trace element, is a com-
ponent of several enzymes in both prokaryotes and eu-
karyotes. These include thioredoxin reductase (Section 28-
3Ae) and the thyroid hormone deiodinases (which
participate in thyroid hormone synthesis; Section 19-1D) in
mammals and three forms of formate dehydrogenases in
E. coli, all of which contain Sec residues. The Sec residues
are ribosomally incorporated into these proteins by a unique
tRNA, tRNA
Sec
, bearing a UCA anticodon that is specified
by a particular (in the mRNA) UGA codon (normally the
opal Stop codon). The Sec–tRNA
Sec
is synthesized by the
aminoacylation of tRNA
Sec
with L-serine by the same
SerRS that charges tRNA
Ser
, followed by the enzymatic
selenylation of the resulting Ser residue.
How does the ribosomal system differentiate a Sec-spec-
ifying UGA codon from a normal opal Stop codon? As we
saw to be the case with Glu–tRNA
Gln
(Section 32-2Cf), EF-
Tu, the elongation factor that conducts most aminoacyl–
tRNAs to the ribosome in a GTP-dependent process, does
not bind Sec–tRNA
Sec
.Instead it is bound by a specific elon-
gation factor named SELB, a homolog of EF-Tu, that in its
complex with GTP is recruited to a ribosomally bound
mRNA stem–loop structure in the selenoprotein coding re-
gion on the 3¿ side of the UGA codon specifying Sec.
Certain methanogenic (methane-producing) archaea
express the enzyme methylamine methyltransferase, which
contains the amino acid residue pyrrolysine (Pyl), a Lys
with its ε-nitrogen in amide linkage to a pyrroline group:
Unlike post-translationally modified Lys residues, such as
5-hydroxylysine (Hyl; Section 8-2B) and ε-N-acetyllysine
N
OC
C
O
CH
NH
CH
2
CH
2
CH
2
CH
2
CH
3
NH
The pyrrolysine (Pyl) residue
The selenocysteine
(Sec) residue
CH CH
2
NH
Se
C
H
O
Section 32-2. Transfer RNA and Its Aminoacylation 1361
JWCL281_c32_1338-1428.qxd 9/7/10 2:22 PM Page 1361