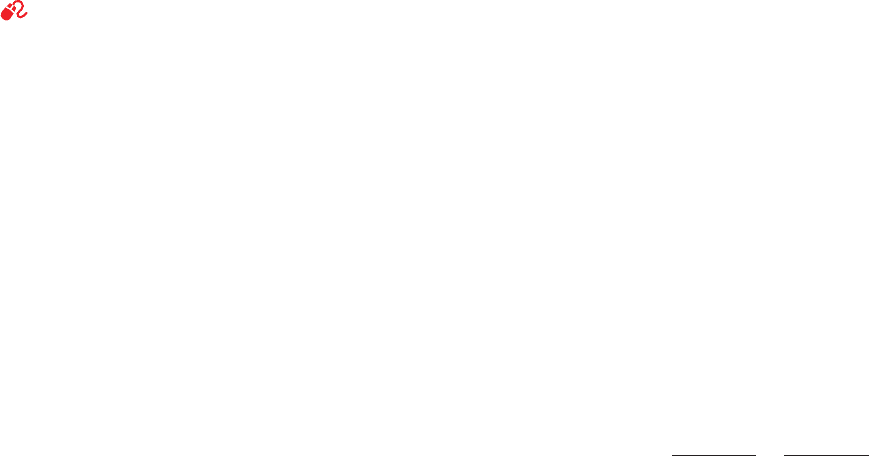
promoter DNA.The structures of ␣CTD
CAP,DNA
and ␣CTD-
CAP
and their interactions with DNA are nearly identical,
thereby suggesting that they are representative of the inter-
action of an ␣CTD with an UP element.
D. Sequence-Specific Protein–DNA Interactions
Since genetic expression is controlled by proteins such as
CAP and lac repressor, an important issue in the study of
gene regulation is how these proteins recognize their target
base sequences on DNA. Sequence-specific DNA-binding
proteins generally do not disrupt the base pairs of the
duplex DNA to which they bind. Consequently, these
proteins can only discriminate among the four base pairs
(A ⴢ T, T ⴢ A, G ⴢ C, and C ⴢ G) according to the functional
groups of these base pairs that project into DNA’s major
and minor grooves. An inspection of Fig. 5-12 reveals that
the groups exposed in the major groove have a greater
variation in their types and arrangements than do those
that are exposed in the minor groove. Indeed, the positions
of the hydrogen bonding acceptors in the major groove
vary with both the identity and orientation of the base pair,
whereas in the minor groove they are largely sequence
independent. Moreover, the ⬃5-Å-wide and ⬃8-Å-deep
minor groove of canonical (ideal) B-DNA is too narrow to
admit protein structural elements such as an ␣ helix,
whereas its ⬃12-Å-wide and ⬃8-Å-deep major groove can
do so. Thus, in the absence of major conformational
changes to B-DNA, it would be expected that proteins
could more readily differentiate base sequences from its
major groove than from its minor groove. We shall see
below that this is, in fact, the case.
a. The Helix–Turn–Helix Motif Is a Common DNA
Recognition Element in Prokaryotes
See Guided Exploration 30: Transcription factor–DNA interactions
The CAP dimer’s two symmetrically disposed F helices
protrude from the protein surface in such a way that they
fit into successive major grooves of B-DNA (Fig. 31-31).
CAP’s E and F helices form a helix–turn–helix (HTH)
motif (supersecondary structure) that conformationally re-
sembles analogous HTH motifs in numerous other prokary-
otic repressors of known X-ray and NMR structure, includ-
ing the lac repressor, the E. coli trp repressor (Section
31-3G), and the cI repressors and Cro proteins from bac-
teriophages and 434 (Section 33-3D). HTH motifs are
⬃20-residue polypeptide segments that form two ␣ helices
which cross at ⬃120° (Fig. 31-31c). They occur as compo-
nents of domains that otherwise have widely varying struc-
tures, although all of them bind DNA. Note that HTH mo-
tifs are structurally stable only when they are components
of larger proteins.
The X-ray and NMR structures of a number of
protein–DNA complexes (see below) indicate that DNA-
binding proteins containing an HTH motif associate with
their target base pairs mainly via the side chains extending
from the second helix of the HTH motif, the so-called recog-
nition helix (helix F in CAP, E in trp repressor, and ␣3 in
the phage proteins). Indeed, replacing the outward-facing
residues of the 434 repressor’s recognition helix with the
corresponding residues of the related bacteriophage P22
yields a hybrid repressor that binds to P22 operators but
not to those of 434. Moreover, the HTH motifs in all these
proteins have amino acid sequences that are similar to each
other and to polypeptide segments in numerous other
prokaryotic DNA-binding proteins, including lac repres-
sor. Evidently, these proteins are evolutionarily related and
bind their target DNAs in a similar manner.
How does the recognition helix recognize its target se-
quence? Since each base pair presents a different and pre-
sumably readily differentiated constellation of hydrogen
bonding groups in DNA’s major groove, it seemed likely
that there would be a simple correspondence, analogous to
Watson–Crick base pairing, between the amino acid
residues of the recognition helix and the bases they contact
in forming sequence-specific associations.The above X-ray
structures, however, indicate this idea to be incorrect.
Rather, base sequence recognition arises from complex
structural interactions. For instance:
1. The X-ray structures of the 48% identical N-termi-
nal domain of 434 repressor (residues 1–69) and the en-
tire 71-residue 434 Cro protein in their complexes with
the identical 20-bp target DNA (the expression of phage
434 is regulated through the differential binding of these
proteins to the same DNA segments; Section 33-3Db)
were both determined by Stephen Harrison. Both ho-
modimeric proteins, as seen for CAP (Fig. 31-31), associ-
ate with the DNA in a 2-fold symmetric manner with their
recognition helices bound in successive turns of the
DNA’s major groove (Figs. 31-32 and 31-33). In both com-
plexes, the protein closely conforms to the DNA surface
and interacts with its paired bases and sugar–phosphate
chains through elaborate networks of hydrogen bonds, salt
bridges, and van der Waals contacts. Nevertheless, the de-
tailed geometries of these associations are significantly
different. In the repressor–DNA complex (Fig. 31-32), the
DNA bends around the protein in an arc of radius ⬃65 Å
which compresses the minor groove by ⬃2.5 Å near its
center (between the two protein monomers) and widens
it by ⬃2.5 Å toward its ends. In contrast, the DNA in com-
plex with Cro (Fig. 31-33), although also bent, is nearly
straight at its center and has a less compressed minor
groove (compare Figs. 31-32a and 31-33a). This explains
why the simultaneous replacement of three residues in
the repressor’s recognition helix with those occurring in
Cro does not cause the resulting hybrid protein to bind
DNA with Cro-like affinity: The different conformations
of the DNA in the repressor and Cro complexes prevents
any particular side chain from interacting identically with
the DNA in the two complexes.
2. Paul Sigler determined the X-ray structure of E. coli
trp repressor in complex with a DNA containing an 18-bp
palindrome (TGTA
CTAGTTAACTAGTAC, where the
trp repressor’s target sequence is underlined) that closely
resembles the trp operator (Section 31-3G). The dimeric
protein’s recognition helices bind, as expected, in succes-
sive major grooves of the DNA, each in contact with an
1288 Chapter 31. Transcription
JWCL281_c31_1260-1337.qxd 8/11/10 9:48 PM Page 1288