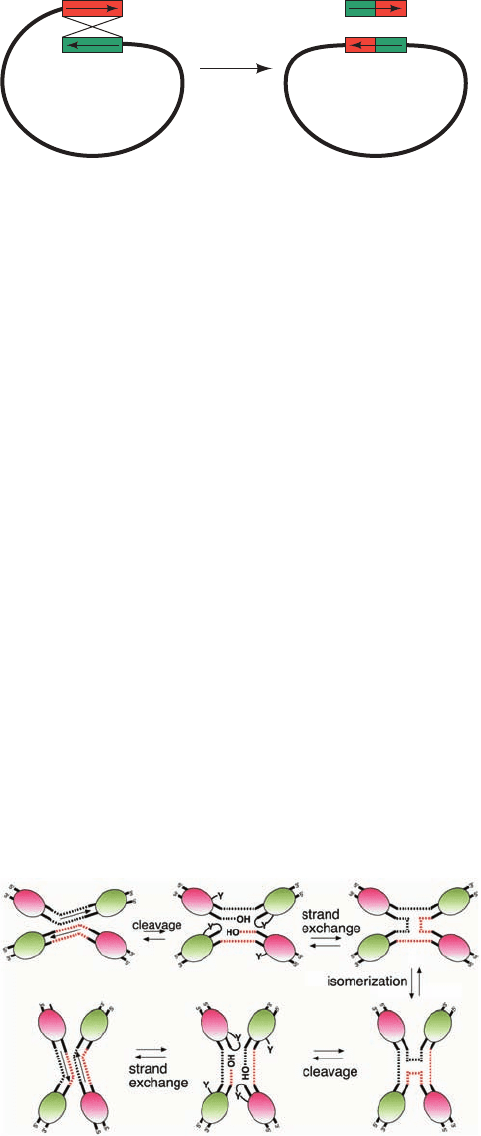
which is encoded by bacteriophage P1, is to mediate the
site-specific recombination between these two loxP sites so
as to circularize the linear DNA (Fig. 30-96).
The loxP site is palindromic except for its central 8-bp
crossover region, which confers directionality on the site.
In carrying out the recombination reaction, the 343-residue
Cre subunits form a homotetramer that binds two loxP sites
in an antiparallel orientation, with each Cre subunit binding
half of a loxP site. Then, as is diagrammed in Fig. 30-97,
oppositely located Cre subunits catalyze single-strand scis-
sions on the 5¿ side of the crossover region on one strand of
each of the two dsDNAs. This occurs through the nucle-
ophilic attack of each of these active Cre subunit’s con-
served Tyr 324 residues on the DNA’s scissile phosphoester
bond to yield a 3¿-phosphoTyr intermediate on one side of
the cleaved bond and a free 5¿-OH group on the other side
(as similarly occurs in the reactions catalyzed by type IB
topoisomerases; Section 29-3Cc). Each of the liberated 5¿-
OH groups then nucleophilically attacks the 3¿-phospho-
Tyr group on the opposite duplex to form a Holliday junc-
tion, thereby releasing the Tyr residues. The Holliday
junction is resolved into two recombined dsDNAs when
the two Cre subunits that had not yet participated in the re-
action mediate the same cleavage and strand exchange re-
actions on the two heretofore unreacted single strands.This
latter process must be preceded by a structural rearrange-
ment (isomerization) of the Cre tetramer that positions the
catalytic Tyr residues in the latter pair of subunits to partic-
ipate in the reaction while those in the former pair of sub-
units are similarly removed from the scene of the action.
Note that this mechanism differs from that mediated by
serine recombinases in that the latter cleave all four DNA
strands prior to initiating their exchange and hence do not
have a Holliday junction intermediate (Section 30-6Bd).
The X-ray structures of Cre tetramers in their complexes
with several loxP model DNAs, determined by Gregory Van
Duyne, have helped elucidate its mechanism.When the DNA
had a single-strand nick past the second nucleotide from the
5¿ end of the crossover region, Cre-catalyzed strand scission
yielded a free nucleotide (a CMP) that diffused away. Since
this nucleotide contained the otherwise reactive 5¿-OH
group, the 3¿-phosphoTyr intermediate was irreversibly
trapped, that is, Cre could not carry out the strand exchange
reaction in Fig. 30-97 (this nicked DNA is a suicide substrate
for Cre; Section 28-3Bc).The X-ray structure of the Cre com-
plex of this nicked DNA confirmed the presence of the 3¿-
phosphoTyr intermediate and indicated, through model
building, that the 5¿-OH group on the missing CMP residue
would be well positioned to nucleophilically attack the 3¿-
phosphoTyr bond on the opposite strand (Fig. 30-98a). Note
that this complex is only 2-fold symmetric although its four
Cre subunits and much of the DNA are related by pseudo-4-
fold symmetry. When the DNA was, instead, an immobile
Holiday junction (Fig. 30-98b),the complex was also pseudo-
4-fold symmetric with the single strands that had crossed
over noticeably kinked at their centers. These structures re-
vealed that the conformational changes necessary to carry
out the strand exchange and isomerization reactions (Fig. 30-
97) required surprisingly small movements on the part of the
Cre subunits and that only the sugar–phosphate backbones
of the strand-exchanged nucleotides needed to move in or-
der to form the Holliday junction.
h. Most Transpositions in Eukaryotes Involve
RNA Intermediates
Transposons similar to those in prokaryotes also occur in
eukaryotes, including yeast, maize, Drosophila, and humans.
In fact, ⬃3% of the human genome consists of DNA-based
transposons although, in most cases, their sequences have
mutated so as to render them inactive, that is, these trans-
posons are evolutionary fossils. However, many eukaryotic
transposons exhibit little similarity to those of prokaryotes.
Rather, their base sequences resemble those of retroviruses
(see below), which suggests that these transposons are de-
generate retroviruses. The transposition of these so-called
retrotransposons occurs via a pathway that resembles the
replication of retroviral DNA (Section 15-4C): (1) their tran-
scription to RNA, (2) the reverse transcriptase–mediated
copying of this RNA to cDNA (Section 30-4C), and (3) the
largely random insertion of this DNA into the host
1244 Chapter 30. DNA Replication, Repair, and Recombination
Figure 30-96 The circularization of linear bacteriophage P1
DNA. This occurs through the Cre-mediated site-specific
recombination between its two terminally located loxP sites (red
and green) to yield its lysogenic plasmid.
Figure 30-97 The mechanism of Cre–loxP site-specific
recombination. The dashed lines represent the nonpalindromic
crossover regions of the loxP sites. The green and magenta Cre
subunits are active for cleavage in the top and bottom parts of
the diagram, respectively, with their roles being switched by the
isomerization step. Note that the mechanism does not require
branch migration of the Holliday junction intermediate.
[Courtesy of Gregory Van Duyne, University of Pennsylvania
School of Medicine.]
Bacteriophage P1
linear
Bacteriophage P1
lysogenic plasmid
Cre
+
LoxP LoxP
LoxP LoxP
JWCL281_c30_1173-1259.qxd 8/10/10 9:13 PM Page 1244