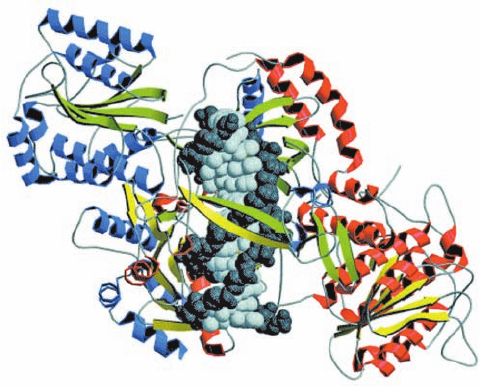
common to all known DNA polymerases (although their
orders differ in the sequences of the different families of
DNA polymerases) and, in addition, has a C-terminal do-
main unique to Y-family DNA polymerases that has been
dubbed the “little finger” domain. The enzyme, as ex-
pected, has incorporated a ddA residue at the 3¿ end of the
primer and, in addition, binds a ddADP in base-paired
complex to the new template T. The little finger domain
binds in the major groove of the DNA. However, the fin-
gers and thumb domains are small and stubby compared
to those of replicative DNA polymerases such as Klentaq1
(Fig. 30-9) and pol ␦ (Fig. 30-41), and the residues that con-
tact the base pair in the active site are all Gly and Ala
rather than the Phe, Tyr, and Arg that mainly do so in the
replicative DNA polymerases. Moreover, the bound DNA
is entirely in the B form rather than in the A form at the
active site as occurs in many replicative DNA poly-
merases. Since the minor groove is more accessible in A-
DNA than in B-DNA (Section 29-1B), this suggests that
error-prone DNA polymerases have relatively little facil-
ity to monitor the base-pairing fidelity of the incoming nu-
cleotide. This accounts for the ability of error-prone DNA
polymerases to accommodate distorted template DNA as
well as non-Watson–Crick base pairs at their active sites.
SOS repair is an error-prone and hence mutagenic
process. It is therefore a process of last resort that is only
initiated ⬃50 min after SOS induction if the DNA has not
already been repaired by other means. Yet, DNA damage
that normally activates the SOS response is nonmutagenic
in the recA
⫺
E. coli that survive.This is, as we saw, because
bypass DNA polymerases will replicate over a DNA lesion
even when there is no information as to which bases were
originally present. Indeed, most mutations in E. coli arise
from the actions of the SOS repair system, which is there-
fore a testimonial to the proposition that survival with a
chance of loss of function (and the possible gain of new
ones) is advantageous, in the Darwinian sense, over death,
although only a small fraction of cells actually survive this
process. It has therefore been suggested that, under condi-
tions of environmental stress, the SOS system functions to
increase the rate of mutation so as to increase the rate at
which the E. coli adapt to the new conditions. Finally, it
should be noted that the eukaryotic pols , , and , all Y-
family members, and pol , an X-family member, are impli-
cated in TLS and that pol , the product of the XPV gene,
is defective in the XPV form of xeroderma pigmentosum
(Section 30-5Bb).
E. Double-Strand Break Repair
Double-strand breaks (DSBs) in DNA are produced when
a replication fork encounters a nick and by the reactive
oxygen species (ROS) by-products of oxidative metabo-
lism and ionizing radiation (which also produces ROS). In
fact, around 5 to 10% of dividing cells in culture exhibit at
least one chromosome break at any given time. Moreover,
DSBs are normal intermediates in certain specialized cel-
lular processes such as recombination during meiosis (Sec-
tion 1-4Ab) and V(D)J recombination in lymphoid cells,
which helps generate the vast diversity of antigen-binding
sites in antibodies and T-cell receptors (Section 35-2C).
Unrepaired or misrepaired DSBs can be lethal to cells or
cause chromosomal aberrations that may lead to cancer.
Hence, the efficient repair of DSBs is essential for cell via-
bility and genomic integrity.
Cells have two general modes to repair DSBs: recombi-
nation repair, which only occurs during the late S and G
2
phases of the cell cycle (when sister chromatids are present
to serve as templates), and nonhomologous end-joining
(NHEJ), which functions throughout the cell cycle. Here
we discuss NHEJ, a process which, as its name implies, di-
rectly rejoins DSBs. The recombination repair of DSBs is
discussed in Section 30-6Ag.
In NHEJ, the broken ends of the DSB must be aligned,
its frayed ends trimmed and/or filled in, and their strands
ligated.The core NHEJ machinery in eukaryotes includes
the DNA end-binding protein Ku (a heterodimer of ho-
mologous 70- and 83-kD subunits, Ku70 and Ku80), DNA
ligase IV, and the accessory protein Xrcc4. Ku, an abun-
dant nuclear protein, binds to a DSB, whether blunt or
with an overhang, and hence appears to be the cell’s pri-
mary DSB sensor. The X-ray structure of Ku in complex
with a 14-bp DNA, determined by Jonathan Goldberg, re-
veals that the protein cradles the dsDNA segment along
its entire length and encircles its central ⬃3 bp segment
(Fig. 30-64). The protein ring is also present in the closely
Section 30-5. Repair of DNA 1223
Figure 30-64 X-ray structure of human Ku protein in complex
with DNA containing 14 bp. The subunits of Ku70 (red helices
and yellow strands) and Ku80 (blue helices and green strands) are
viewed along the pseudo-2-fold axis relating them.The DNA,
viewed with its DSB pointing upward, is drawn in space-filling
form with its sugar–phosphate backbone dark gray and its base
pairs light gray. Note that the DNA is surrounded by a ring of
protein. [Courtesy of John Tainer,The Scripps Research Institute,
La Jolla, California. Based on an X-ray structure by Jonathan
Goldberg, Memorial Sloan-Kettering Cancer Center, New York,
New York. PDBid 1JEY.]
JWCL281_c30_1173-1259.qxd 8/10/10 9:12 PM Page 1223