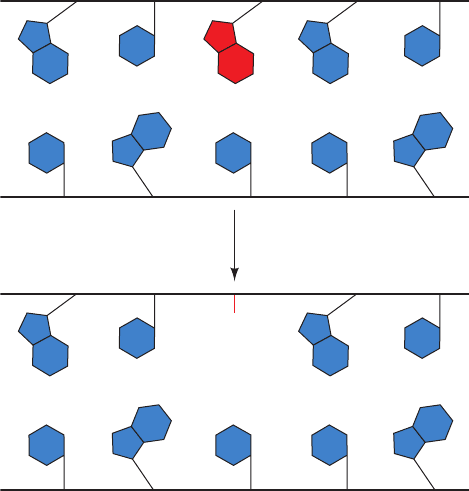
Cockayne syndrome (CS) is an inherited disease caused
by defective TCR. Individuals with CS are hypersensitive to
UV radiation (although they have a normal incidence of
skin cancer) and exhibit stunted growth as well as neuro-
logical dysfunction due to neuron demyelination leading to
death in childhood. CS is most often caused by mutations
in two complementation groups, CSA and CSB, although
certain defects in XPB, XPD, and XPG can also cause CS
in addition to XP.
The retarded development typical of XPB and XPD de-
fects and perhaps the demyelination that occurs in CS appear
to be due more to impaired transcription than to defective
NER. This is explained by the fact that the DNA helicases
XPB and XPD are subunits of the ten-subunit eukaryotic
transcription factor TFIIH, whose proper functioning is re-
quired for transcription initiation by RNA polymerase II
(Section 34-3Bb) as well as NER and TCR. A eukaryotic
RNA polymerase that is stalled at a DNA lesion is recog-
nized by CSB, which then recruits the other TCR compo-
nents. CSA interacts with both CSB and the p44 subunit of
TFIIH. Once the damage has been repaired, the RNA poly-
merase resumes transcription.Thus, in CS, RNA polymerase
molecules become permanently stalled on DNA lesions.
TCR also occurs in bacteria. For example, in E. coli, the
protein TCRF (for transcription repair couping factor; also
known as Mfd protein, for mutation frequency decline) is
an ATP-powered DNA translocase that displaces stalled
RNA polymerase from the damaged template strand, fol-
lowing which TCRF recruits the proteins of the UvrABC
system to the damage site. The repaired gene is then tran-
scribed from its beginning. Since prokaryotic genes are
much shorter than eukaryotic genes (most of which con-
tain several long noncoding segments known as introns;
Section 31-4Ac), this is a more efficient use of resources
than generating the complex machinery necessary to
restart transcription as occurs in eukaryotes.
c. Base Excision Repair
DNA bases are modified by reactions that occur under
normal physiological conditions as well as through the action
of environmental agents. For example, adenine and cytosine
residues spontaneously deaminate at finite rates to yield hy-
poxanthine and uracil residues, respectively. S-Adenosylme-
thionine (SAM), a common metabolic methylating agent
(Section 26-3Ea), occasionally nonenzymatically methylates
a base to form derivatives such as 3-methyladenine and 7-
methylguanine residues (Fig. 30-54). Ionizing radiation can
promote ring opening reactions in bases. Such changes mod-
ify or eliminate base pairing properties.
DNA containing a damaged base may be restored to its
native state through base excision repair (BER). Cells con-
tain a variety of DNA glycosylases that each cleave the gly-
cosidic bond of a corresponding specific type of altered nu-
cleotide (Fig. 30-59), thereby leaving a deoxyribose residue
in the backbone. Such apurinic or apyrimidinic (AP) sites
(also called abasic sites) are also generated under normal
physiological conditions by the spontaneous hydrolysis of a
glycosidic bond. The deoxyribose residue is then cleaved
on one side by an AP endonuclease, the deoxyribose and
several adjacent residues are removed by the action of a
cellular exonuclease (possibly associated with a DNA poly-
merase), and the gap is filled in and sealed by a DNA poly-
merase and DNA ligase.
d. Uracil in DNA Would Be Highly Mutagenic
For some time after the essential functions of nucleic
acids had been elucidated, there seemed no apparent reason
for nature to go to the considerable metabolic effort of using
thymine in DNA and uracil in RNA when these substances
have virtually identical base pairing properties. This enigma
was solved by the discovery of cytosine’s penchant for con-
version to uracil by deamination, either via spontaneous hy-
drolysis (Fig. 30-54), which is estimated to occur ⬃120 times
per day in each human cell, or by reaction with nitrites (Sec-
tion 32-1Aa). If U were the normal DNA base, the deamina-
tion of C would be highly mutagenic because there would be
no indication of whether the resulting mismatched G ⴢ U
base pair had originally been G ⴢ C or A ⴢ U. Since T is DNA’s
normal base, however, any U in DNA is almost certainly a
deaminated C. U’s that occur in DNA are efficiently excised
by uracil–DNA glycosylase [UDG; also called uracil N-gly-
cosylase (UNG)] and then replaced by C through BER.
UDG also has an important function in DNA replica-
tion. dUTP, an intermediate in dTTP synthesis, is present in
all cells in small amounts (Section 28-3Ba). DNA poly-
merases do not discriminate well between dUTP and dTTP
(recall that DNA polymerases select a base for incorpora-
tion into DNA according to its ability to form a
Watson–Crick-shaped base pair with the template; Section
30-2Aa) so that, despite the low dUTP level that cells main-
tain, newly synthesized DNA contains an occasional U.
These U’s are rapidly replaced by T through BER. How-
ever, since excision occurs more rapidly than repair, all
1218 Chapter 30. DNA Replication, Repair, and Recombination
Figure 30-59 Action of DNA glycosylases. These enzymes
hydrolyze the glycosidic bond of their corresponding altered
base (red) to yield an AP site.
OH
DNA glycosylase
JWCL281_c30_1173-1259.qxd 8/10/10 9:11 PM Page 1218