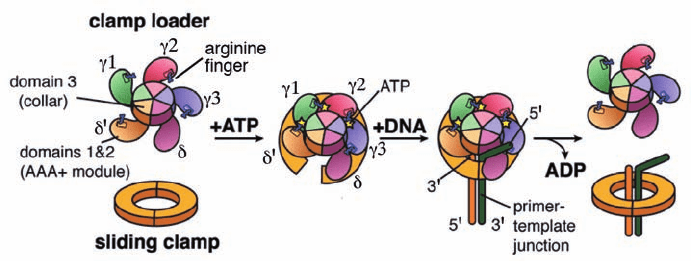
1198 Chapter 30. DNA Replication, Repair, and Recombination
Figure 30-35 Schematic diagram of the clamp loader cycle.
This speculative model is based on a combination of structural
and biochemical information.The “arginine finger,” an Arg side
chain that interacts with the ␥-phosphate group of an ATP bound
tacts between the core and the DNA). Thus once the slid-
ing clamp has been loaded onto the primer–template, the
clamp loader is replaced by the Pol III core, which thereby
blocks the clamp loader from unloading the clamp. Instead,
the clamp loader loads a new clamp onto the lagging strand
template in association with the primer that the primo-
some had synthesized in preparation for the next round of
Okazaki fragment synthesis (Fig. 30-28b).
The X-ray structure of the sliding clamp in complex with
primer–template DNA (Fig. 30-13) indicates that its
ssDNA segment binds to the same site as do the ␣ and ␦
subunits. This may serve to attract the primer–template
DNA to the inside of the open clamp, which in turn may
facilitate the release of the clamp loader and hence the clo-
sure of the sliding clamp. The binding of ssDNA to the
sliding clamp may also prevent it from sliding away before
it can be bound by the ␣ subunit.
When the Pol III core has completed its synthesis of the
Okazaki fragment, that is, when the gap between the two
successively synthesized Okazaki fragments has been re-
duced to a nick, it releases the DNA and the sliding clamp.
The Pol III core then binds to the newly primed template
and its associated clamp (displacing the clamp loader),
where it commences the synthesis of the next Okazaki
fragment. Thus, a series of switches that are activated by
ATP and DNA structure ensure the vectorial progression
of lagging strand replication. Throughout this process, the
Pol III holoenzyme is held at the replication fork by the
leading strand Pol III core, which remains tethered to
the DNA by its associated sliding clamp.
The sliding clamp that remains around the completed
Okazaki fragment probably functions to recruit Pol I and
DNA ligase so as to replace the RNA primer on the previ-
ously synthesized Okazaki fragment with DNA and seal
the remaining nick. However, the sliding clamp must even-
tually be recycled. It was initially assumed that this was the
job of the clamp loader. However, it is now clear that the
release of the sliding clamp from its associated DNA is
largely carried out by free ␦ subunit (the “wrench” in the
clamp loader that cracks apart the  subunits forming the
sliding clamp), which is synthesized in 5-fold excess over
that required to populate the cell’s few clamp loaders.
loader associates with the DNA almost entirely through
contacts with the phosphate groups of the template strand
alone. Thus, this structure is reminiscent of that of the E1
helicase in complex with ssDNA (Section 30-2Ca) with one
of its six subunits missing.
The clamp loader must tightly bind the sliding clamp
prior to its loading on the template DNA but must subse-
quently release the clamp to avoid interfering with its
binding to the Pol III core (␣ε). The structures of the
clamp loader and the –␦ complex, together with a variety
of biochemical evidence, suggest a model of how this
might occur (Fig. 30-35): The binding of ATP to ␥1 (the ␥
subunit that contacts ␦¿) results in a conformational
change that exposes the otherwise occluded ATP-binding
site of ␥2;ATP binding to ␥2 likewise exposes ␥3; and ATP
binding to ␥3 exposes the ␦ subunit’s  interaction ele-
ment, thereby permitting it to bind to a  subunit so as to
spring open the sliding clamp. Primer–template DNA then
inserts itself through the resulting gap in the sliding clamp.
This process is facilitated by the gap between the AAA⫹
domains of ␦ and ␦¿ subunits, which permits the clamp
loader to track the template strand while avoiding contact
with the primer strand. Eventually, - and DNA-stimu-
lated hydrolysis of the bound ATPs releases the  subunit
from the clamp loader, whereon the sliding clamp closes
around the DNA.
The departure of the clamp loader permits the Pol III
core to bind to the sliding clamp. However, when the syn-
thesis of an Okazaki fragment has been completed, the Pol
III core must dissociate from the sliding clamp so that it
can initiate the synthesis of the next Okazaki fragment.
How does this occur?
Pol III’s ␣ subunit binds to the same hydrophobic
pocket on the 
2
sliding clamp as does the ␦ subunit. This
was shown by the observations that the phosphorylation of
a kinase recognition sequence that had been engineered
into the C-terminal segment of  is inhibited by both ␣ and
␦. The  subunit has an ⬃30-fold greater affinity for the ␥
complex in the presence of ATP than it has for the Pol III
core. However, when primer–template DNA is also pres-
ent, this order of affinity is reversed with  preferring to
bind to the Pol III core (possibly due to the additional con-
to a neighboring subunit, is a common feature of AAA⫹
ATPases that form ringlike structures. [Modified from a drawing
by Mike O’Donnell,The Rockefeller University, and John
Kuriyan, University of California at Berkeley.]
JWCL281_c30_1173-1259.qxd 8/10/10 9:11 PM Page 1198