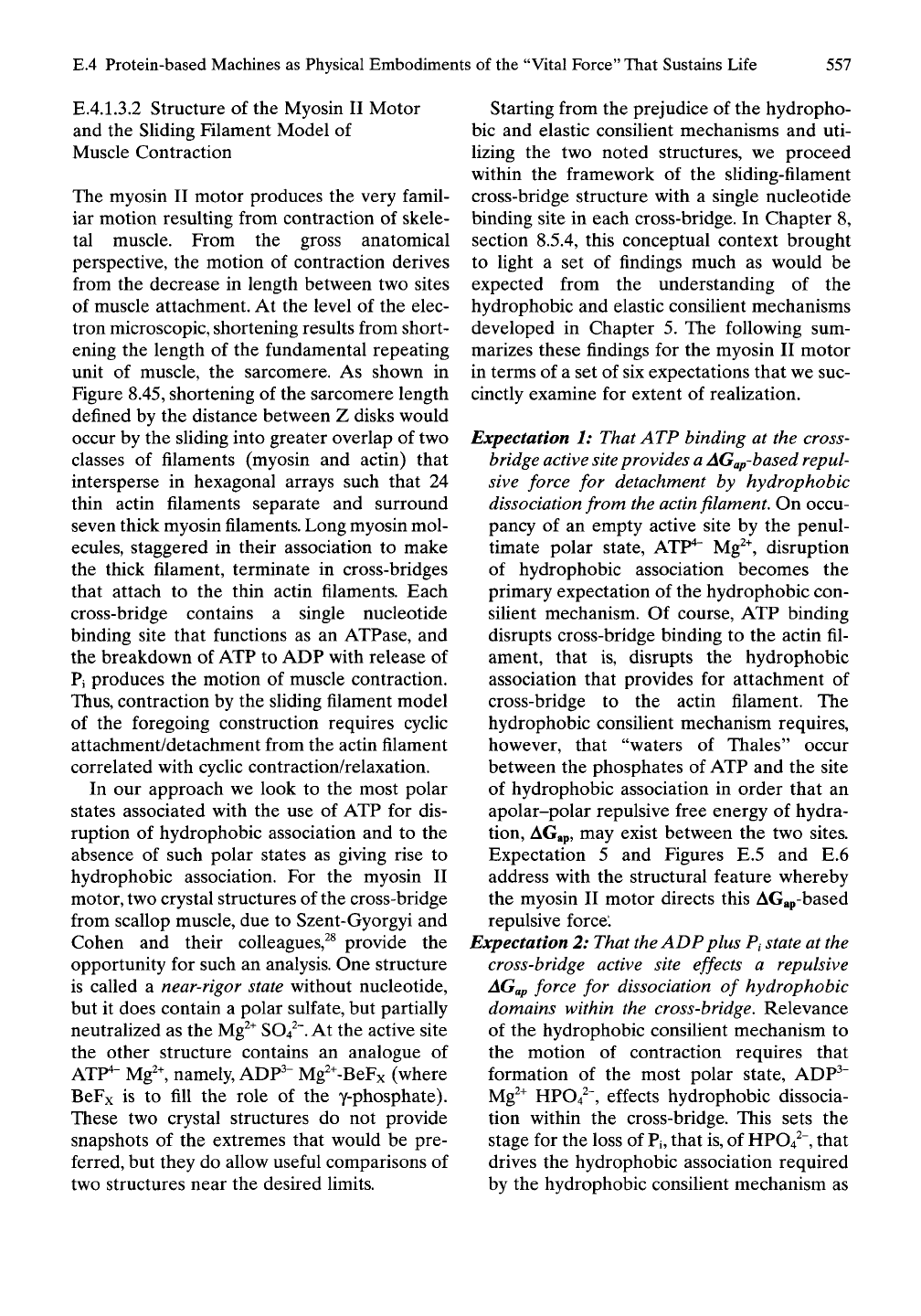
E.4 Protein-based Machines as Physical Embodiments of the "Vital Force" That Sustains Life
557
E.4.1.3.2 Structure of the Myosin II Motor
and the Sliding Filament Model of
Muscle Contraction
The myosin II motor produces the very famil-
iar motion resulting from contraction of skele-
tal muscle. From the gross anatomical
perspective, the motion of contraction derives
from the decrease in length between two sites
of muscle attachment. At the level of the elec-
tron microscopic, shortening results from short-
ening the length of the fundamental repeating
unit of muscle, the sarcomere. As shown in
Figure 8.45, shortening of the sarcomere length
defined by the distance between Z disks would
occur by the sliding into greater overlap of two
classes of filaments (myosin and actin) that
intersperse in hexagonal arrays such that 24
thin actin filaments separate and surround
seven thick myosin filaments. Long myosin mol-
ecules, staggered in their association to make
the thick filament, terminate in cross-bridges
that attach to the thin actin filaments. Each
cross-bridge contains a single nucleotide
binding site that functions as an ATPase, and
the breakdown of ATP to ADP with release of
Pi produces the motion of muscle contraction.
Thus,
contraction by the sliding filament model
of the foregoing construction requires cyclic
attachment/detachment from the actin filament
correlated with cyclic contraction/relaxation.
In our approach we look to the most polar
states associated with the use of ATP for dis-
ruption of hydrophobic association and to the
absence of such polar states as giving rise to
hydrophobic association. For the myosin II
motor, two crystal structures of the cross-bridge
from scallop muscle, due to Szent-Gyorgyi and
Cohen and their coUeagues,^^ provide the
opportunity for such an analysis. One structure
is called a near-rigor state without nucleotide,
but it does contain a polar sulfate, but partially
neutralized as the
Mg^"^
SO/~. At the active site
the other structure contains an analogue of
ATP^-
Mg'% namely, ADP^" Mg^^-BeFx (where
BeFx is to fill the role of the y-phosphate).
These two crystal structures do not provide
snapshots of the extremes that would be pre-
ferred, but they do allow useful comparisons of
two structures near the desired limits.
Starting from the prejudice of the hydropho-
bic and elastic consilient mechanisms and uti-
lizing the two noted structures, we proceed
within the framework of the sliding-filament
cross-bridge structure with a single nucleotide
binding site in each cross-bridge. In Chapter 8,
section 8.5.4, this conceptual context brought
to light a set of findings much as would be
expected from the understanding of the
hydrophobic and elastic consilient mechanisms
developed in Chapter 5. The following sum-
marizes these findings for the myosin II motor
in terms of a set of six expectations that we suc-
cinctly examine for extent of realization.
Expectation 1: That ATP binding at the cross-
bridge active site provides a AGap-based repul-
sive force for detachment by hydrophobic
dissociation from the actin filament. On occu-
pancy of an empty active site by the penul-
timate polar state, ATP"^" Mg^"^, disruption
of hydrophobic association becomes the
primary expectation of the hydrophobic con-
silient mechanism. Of course, ATP binding
disrupts cross-bridge binding to the actin fil-
ament, that is, disrupts the hydrophobic
association that provides for attachment of
cross-bridge to the actin filament. The
hydrophobic consilient mechanism requires,
however, that "waters of Thales" occur
between the phosphates of ATP and the site
of hydrophobic association in order that an
apolar-polar repulsive free energy of hydra-
tion, AGap, may exist between the two sites.
Expectation 5 and Figures E.5 and E.6
address with the structural feature whereby
the myosin II motor directs this AGap-based
repulsive force'.
Expectation 2: That the ADP plus Pi state at the
cross-bridge active site effects a repulsive
AGap force for dissociation of hydrophobic
domains within the cross-bridge. Relevance
of the hydrophobic consihent mechanism to
the motion of contraction requires that
formation of the most polar state, ADP^"
Mg^^ HPO/~, effects hydrophobic dissocia-
tion within the cross-bridge. This sets the
stage for the loss of Pi, that is, of HPO/", that
drives the hydrophobic association required
by the hydrophobic consilient mechanism as