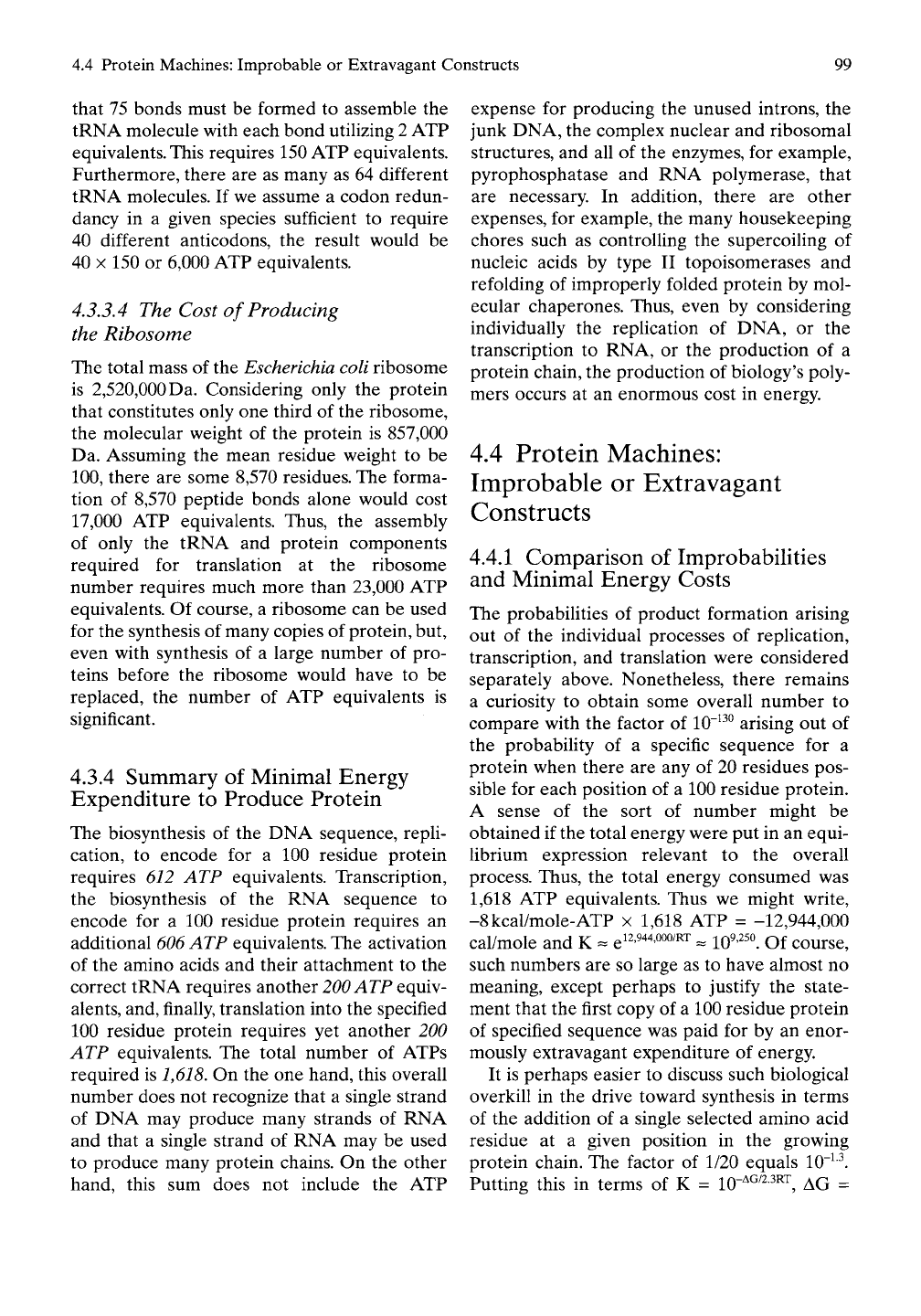
4.4 Protein Machines: Improbable or Extravagant Constructs
99
that 75 bonds must be formed to assemble the
tRNA molecule with each bond utilizing 2 ATP
equivalents. This requires 150 ATP equivalents.
Furthermore, there are as many as 64 different
tRNA molecules. If we assume a codon redun-
dancy in a given species sufficient to require
40 different anticodons, the result would be
40
X
150 or 6,000 ATP equivalents.
4.3.3.4 The Cost of Producing
the Ribosome
The total mass of the Escherichia coli ribosome
is 2,520,000 Da. Considering only the protein
that constitutes only one third of the ribosome,
the molecular weight of the protein is 857,000
Da. Assuming the mean residue weight to be
100,
there are some
8,570
residues. The forma-
tion of
8,570
peptide bonds alone would cost
17,000 ATP equivalents. Thus, the assembly
of only the tRNA and protein components
required for translation at the ribosome
number requires much more than 23,000 ATP
equivalents. Of course, a ribosome can be used
for the synthesis of many copies of protein, but,
even with synthesis of a large number of pro-
teins before the ribosome would have to be
replaced, the number of ATP equivalents is
significant.
4.3.4 Summary of Minimal Energy
Expenditure to Produce Protein
The biosynthesis of the DNA sequence, repli-
cation, to encode for a 100 residue protein
requires 612 ATP equivalents. Transcription,
the biosynthesis of the RNA sequence to
encode for a 100 residue protein requires an
additional 606 ATP equivalents. The activation
of the amino acids and their attachment to the
correct tRNA requires another 200 ATP equiv-
alents,
and, finally, translation into the specified
100 residue protein requires yet another 200
ATP equivalents. The total number of ATPs
required is
1^618.
On the one hand, this overall
number does not recognize that a single strand
of DNA may produce many strands of RNA
and that a single strand of RNA may be used
to produce many protein chains. On the other
hand, this sum does not include the ATP
expense for producing the unused introns, the
junk DNA, the complex nuclear and ribosomal
structures, and all of the enzymes, for example,
pyrophosphatase and RNA polymerase, that
are necessary. In addition, there are other
expenses, for example, the many housekeeping
chores such as controUing the supercoiling of
nucleic acids by type II topoisomerases and
refolding of improperly folded protein by mol-
ecular chaperones. Thus, even by considering
individually the replication of DNA, or the
transcription to RNA, or the production of a
protein chain, the production of biology's poly-
mers occurs at an enormous cost in energy.
4.4 Protein Machines:
Improbable or Extravagant
Constructs
4.4.1 Comparison of Improbabilities
and Minimal Energy Costs
The probabilities of product formation arising
out of the individual processes of replication,
transcription, and translation were considered
separately above. Nonetheless, there remains
a curiosity to obtain some overall number to
compare with the factor of
10"^^^
arising out of
the probability of a specific sequence for a
protein when there are any of 20 residues pos-
sible for each position of a 100 residue protein.
A sense of the sort of number might be
obtained if the total energy were put in an equi-
librium expression relevant to the overall
process. Thus, the total energy consumed was
1,618 ATP equivalents. Thus we might write,
-8kcal/mole-ATP x 1,618 ATP = -12,944,000
cal/mole and K - e^^'^^^'^^^^^^ -
10^'^^^
Of course,
such numbers are so large as to have almost no
meaning, except perhaps to justify the state-
ment that the first copy of a 100 residue protein
of specified sequence was paid for by an enor-
mously extravagant expenditure of energy.
It is perhaps easier to discuss such biological
overkill in the drive toward synthesis in terms
of the addition of a single selected amino acid
residue at a given position in the growing
protein chain. The factor of 1/20 equals
10"^
^.
Putting this in terms of K =
IQ-^^/^^^^,
AG =