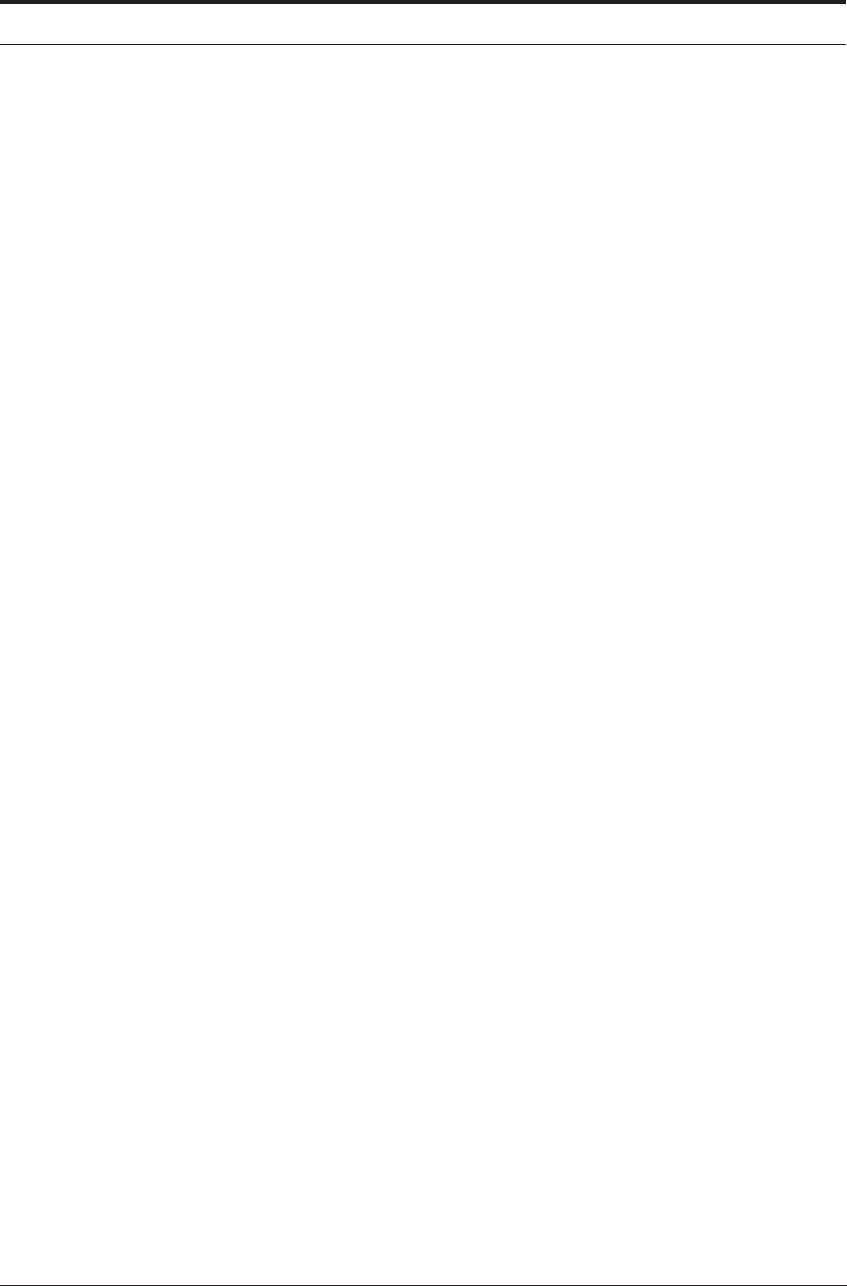
394 MODELING OF METAL CUTTING
before an ABAQUS version 5.4 input file was created. The mesh was oriented in the direction of
the movement between tool and workpiece. The analysis deck created by MSC/PATRAN for
ABAQUS was edited to modify material parameters for use with a “damage/plasticity model
65
and to set the problem up to use a conjugate gradient analysis technique. Post-processing was
performed by ABAQUS.
The “damage/plasticity model”
65
was a material model which accounted for the deviatoric
deformation resulting from the presence of dislocations and dilatational deformation and ensu-
ing failure from the growth of voids. This model allowed the calculation of accumulated damage
in a finite element. The capability of the ABAQUS finite element code was used to turn finite
elements “off” when a user-defined parameter level was reached in each element. This elimi-
nated elements which otherwise would have had large distortions (causing convergence prob-
lems in the analysis) but which physically had failed or had lost their load carrying capability.
This adequately represented the cutting/failure/chip formation mechanisms without requiring
remeshing or mesh adapting. As in all the experiments reported in Chapter 4, the highest normal
stresses were localized at the cutting tip. The analytical stresses in the cutting direction com-
pared well with the corresponding experimental stresses Figure 12.13. The most notable differ-
ence between two stress profiles was that the spatial gradient of the stress magnitude decreased
more quickly with distance in the finite element analysis. Kistler attributed this difference to the
effects in the chip tool contact area. The FEA assumed frictionless conditions for convenience,
and calculated a contact length of 0.58mm. In the experiments, the contact length was 0.85mm.
These different values of contact length almost certainly account for the differences in the nor-
mal stress profile.
12.5.8 Case study III: cutting temperature of ceramic tools
El-Wardany, Mohammed and Elbestawi carried out investigations into the different factors
which influence the temperature distribution in A1
2
0
3
-TiC ceramic tool rake face during
machining of difficult-to-cut materials, such as case hardened AISI 1552 steel (60-65 Rc) and
nickel-based superalloys (e.g. Inconel 718). A commercially available finite element program
(ALGOR) was used to calculate the temperature distributions.
66
Temperature measurements on
the tool rake face were performed using a thermocouple based technique and the results com-
pared with the finite element analysis. Experiments were then performed to study the effect of
cutting parameters, different tool geometries, tool conditions, and workpiece materials on the
cutting edge temperatures.
Of particular interest, it was found that there is an optimum value of rake angle where the cut-
ting edge temperature was minimum. Details are given in Figure 12.14. Initially the edge tem-
perature reduced with a “more negative rake angle” from -6° to -20°; but beyond -20°, to even
more negative values, the temperatures increased again. The same trend was obtained even for a
higher feed of 0.15 mm/rev. El-Wardany et al provide the following explanation: At -6° rake
angle the “air-gap” between the tool’s clearance face and the cut surface is relatively small.
Moving from -6° to -20° rake angle, the tool gets “rotated/lifted up” away from the surface and
there is a greater chance of cooling by convection. But eventually, the shearing of the material in
the secondary shear zone gets to be so intense with more aggressively negative rake angles, that
the overall heat generated is larger and temperatures rise again.