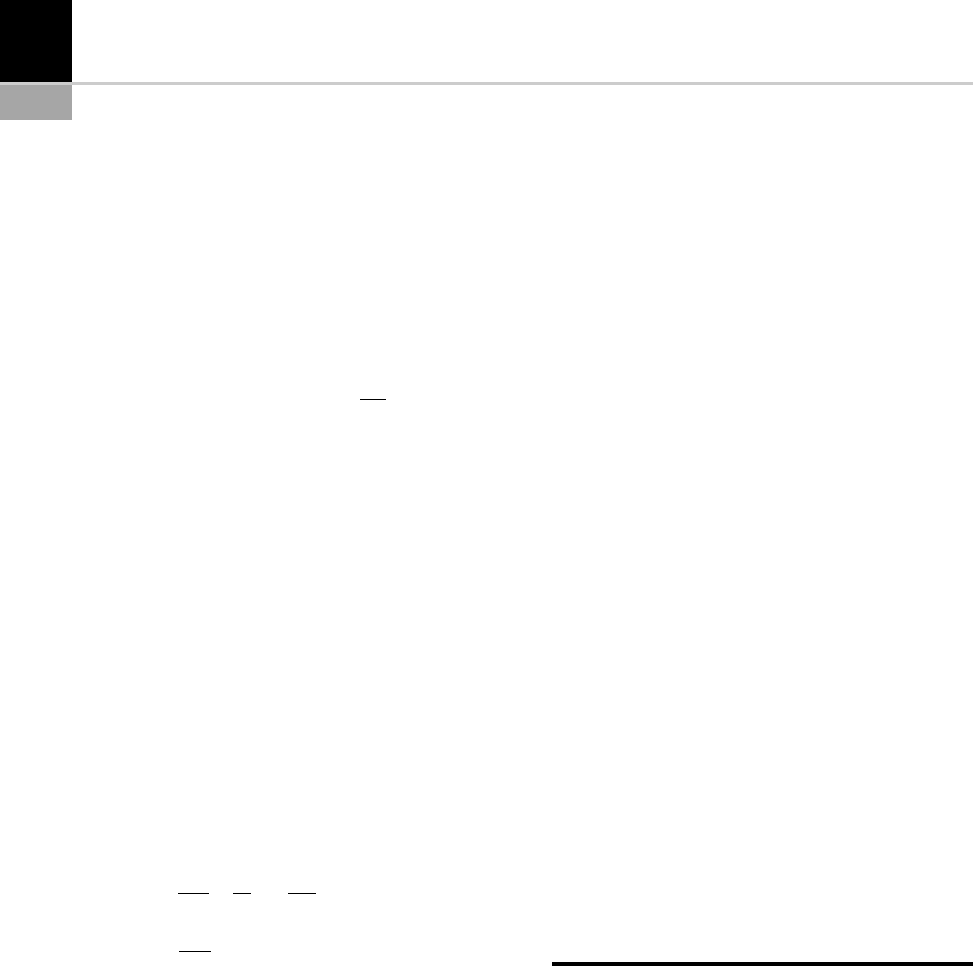
absorption of the photons, and the thermaliza-
tion of the electron and phonon system, are
much smaller than the pulse duration, resulting
in the description of heating by one tempera-
ture for the electron and the phonon system.
Ablation affects the subsystems as an instanta-
neous process. The threshold fluence for
ablation (fluence = energy per area) scales with
the square root of the pulse duration and a
thermal penetration depth can be defined as:
d
therm
¼ 2
ffiffiffiffiffiffiffiffi
kt
p
c
p
r
s
ð3Þ
depending on the thermal property of matter,
with k the thermal conductivity, c
p
the heat
capacity,
_
r the density and t
p
the pulse dura-
tion of the applied radiation. The thermal
penetration depth
_
d defines the region beyond
the focus diameter (tool diameter), which can
be thermally modified, such as by the amor-
phization of crystalline substrate. This regime
is called the heat affected zone (HAZ). The
processing diameter is given by 2(w
0
+ d).
2. Pulse duration smaller than the electron–pho-
non relaxation time
_
t < t
ep
. For t < t
ep
the
processes within the electron and the phonon
system are decoupled. The temperature devel-
opment for these systems are described by a
two-temperature model representing two cou-
pled differential equations:
C
e
dT
e
dt
¼
L
Lz
k
e
LT
e
Lz
þ S m T
e
T
p
C
p
dT
p
dt
¼ m T
e
T
p
ð4Þ
C
e
and C
p
represent the heat capacities of the
electron and the phonon system, k the heat
conductivity of the electron system, S the
applied optical energy, m the electron–phonon
coupling constant and T
e
and T
p
the tempera-
tures of the two systems. Ablation of materials
is characterized by negligible melt and small
mechanical load of the irradiated region. The
processing diameter is comparable to the
focus diameter.
In nanosecond laser processing this energy
transfer occurs during the duration of the
laser pulse, whereas in picosecond laser pro-
cessing the energy transfer occurs after a
certain interaction time. For metals, this
transfer time is generally in the range of some
picoseconds. In this case, the material is
heated up after the end of the laser pulse, so
that there is no interaction of the photons
with melted and evaporated material. The
res u l t is a much more accurate ablation
because the ablation is mainly due to vapori-
zation of the material and not by melt expulsion.
The second reason for the use of picosecond
pulse durations in laser ablation is the very
high intensity of the pulses. With peak intensi-
ties of more than 10
10
W/cm
2
all materials are
vaporized rather than merely melted. As a con-
sequence of these high intensities, the ablation
rate per pulse is quite low, because for vapori-
zation an excessive amount of energy is needed.
In Fig. 4-3(a) direct comparison of laser ablated
steel with nanosecond pulses and picosecond
pulses is shown. It can be seen c learly that in
nanosecond ablation the residual melt after
ablation is much stronger and the geometry
is affected by melt resolidification. In pico-
second ablation almost no melt can be found
in the ablation area and clean surfaces with a
surface r oughness of <0.5 mm can be produced
by laser ablation.
PROCESS PRINCIPLES FOR LASER
ABLATION
Micro-structuring with laser radiation adopts
one of the follow ing techniques:
*
the mask technique
*
the scribing techni que.
The mask technique is appropriate for 2
1
/
2
dimensional structuring, for example appli-
cation in lithography and the generation of
micro-fluidic systems and nano-scaled optical
devices such as gratings. The scribing technique
is ad opted for full 3D struct uring and is com-
parable to mechanical milling.
62 CHAPTER 4 Laser Micro-Structuring