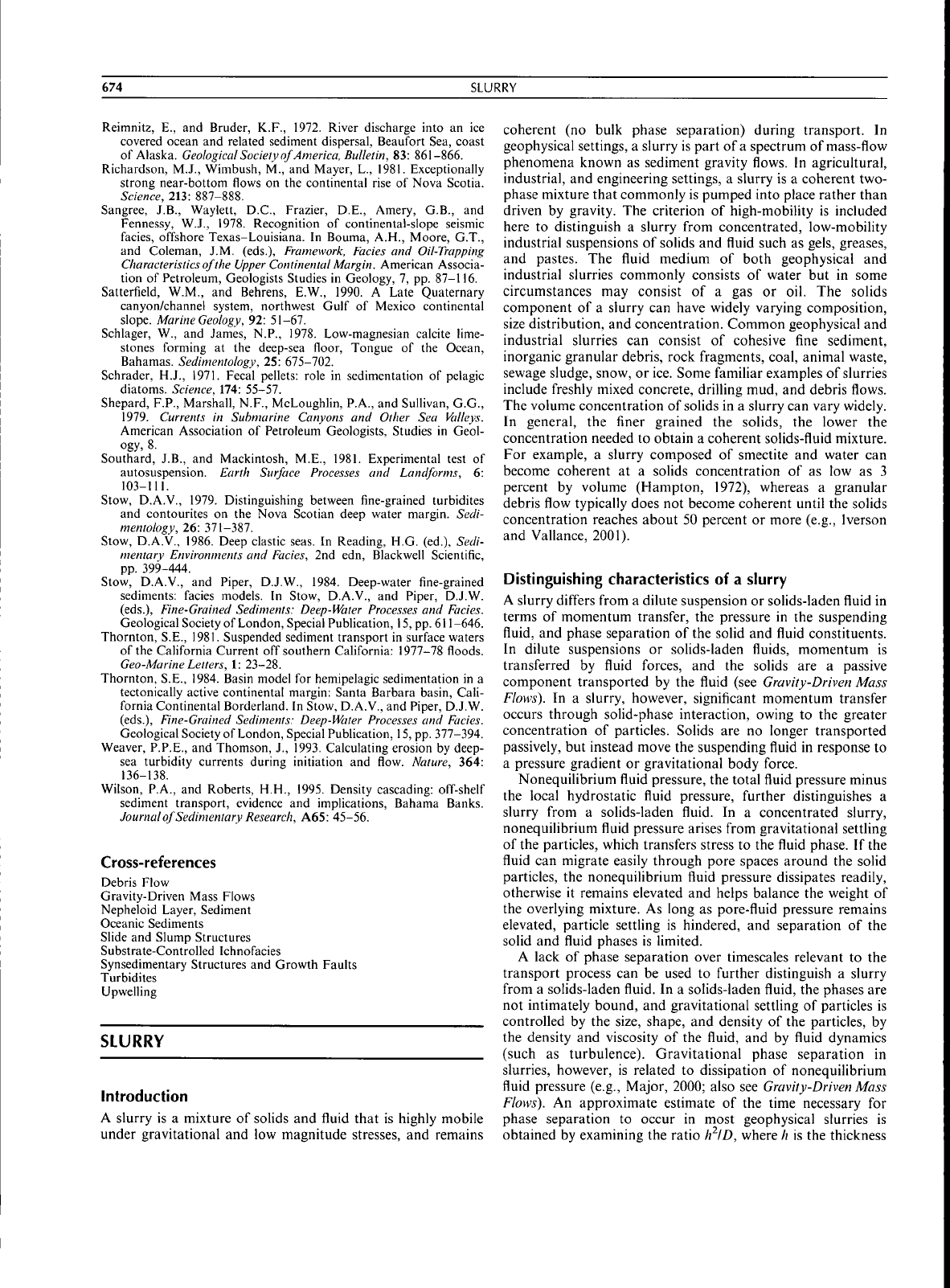
674
SLURRY
Reimnitz, E., and Bruder, K.F., 1972. River discharge into an ice
covered ocean and related sediment dispersal, Beaufort Sea, coast
of Alaska.
Geological
Society of America, Bulletin, 83: 861-866.
Richardson, M.J., Wimbush, M., and Mayer, L., 1981. Exceptionally
strong near-bottom flows on the continental rise of Nova Scotia.
Science, 213: 887-888.
Sangree, J.B., Waylett, D.C, Frazier, D.E., Amery, G.B., and
Fennessy, W.J., 1978. Recognition of continental-slope seismic
facies,
offshore Texas-Louisiana. In Bouma, A.H., Moore, G.T.,
and Coleman, J.M. (eds.). Framework, Facies and Oil-Trapping
Characteristics of the
Upper
Continental Margin. American Associa-
tion of Petroleum, Geologists Studies in Geology, 7, pp. 87-116.
Satterfield, W.M., and Behrens, E.W., 1990. A Late Quaternary
canyon/channel system, northwest Gulf of Mexico continental
slope. Marine Geology, 92: 51-67.
Schlager, W., and James, N.P., 1978. Low-magnesian calcite lime-
stones forming at the deep-sea floor. Tongue of the Ocean,
Bahamas. Sedimentology, 25: 675-702.
Schrader, H.J., 1971. Fecal pellets: role in sedimentation of pelagic
diatoms. Science, 174: 55-57.
Shepard, F.P., Marshall, N.F., McLoughlin, P.A., and Sullivan, G.G.,
1979.
Currents in Submarine Canyons and Other Sea Valleys.
American Association of Petroleum Geologists, Studies in Geol-
ogy, 8.
Southard, J.B., and Mackintosh, M.E., 1981. Experimental test of
autosuspension. Earth Surface Processes and Landforms, 6:
103-111.
Stow, D.A.V., 1979. Distinguishing between fine-grained turbidites
and contourites on the Nova Scotian deep water margin. Sedi-
mentology, 26: 371-387.
Stow, D.A.V., 1986. Deep clastic seas. In Reading, H.G. (ed.). Sedi-
mentary Enviromnents and Facies, 2nd edn, Blackwell Scientific,
pp.
399-444.
Stow, D.A.V., and Piper, D.J.W., 1984. Deep-water fine-grained
sediments: facies models. In Stow, D.A.V., and Piper, D.J.W.
(eds.),
Fine-Grained Sediments: Deep-Water Processes and Facies.
Geological Society of London, Special Publication, 15, pp. 611-646.
Thornton, S.E., 1981. Suspended sediment transport in surface waters
of the California Current off southern Cahfornia: 1977-78 floods.
Geo-Marine Letters, 1: 23-28.
Thornton, S.E., 1984. Basin model for hemipelagie sedimentation in a
tectonically active continental margin: Santa Barbara basin, Cali-
fornia Continental Borderland. In Stow, D.A.V., and Piper, D.J.W.
(eds.),
Fine-Grained Sediments: Deep-Water Processes and Facies.
Geological Society of London, Special Publication, 15, pp. 377-394.
Weaver, P.P.E., and Thomson, J., 1993. Calculating erosion by deep-
sea turbidity currents during initiation and flow. Nature, 364:
136-138.
Wilson, P.A., and Roberts, H.H., 1995. Density cascading: off-shelf
sediment transport, evidence and implications, Bahama Banks.
Journal of Sedimentary Research, A65: 45-56.
Cross-references
Debris Flow
Gravity-Driven Mass Flows
Nepheloid Layer, Sediment
Oceanic Sediments
Slide and Slump Struetures
Substrate-Controlled Ichnofacies
Synsedimentary Structures and Growth Faults
Turbidites
Upwelling
SLURRY
Introduction
A slurry is a mixture of solids and fluid that is highly mobile
under gravitational and low magnitude stresses, and remains
coherent (no bulk phase separation) during transport. In
geophysical settings, a slurry is part ofa spectrum of mass-flow
phenomena known as sediment gravity flows. In agricultural,
industrial, and engineering settings, a slurry is a coherent two-
phase mixture that commonly is pumped into place rather than
driven by gravity. The criterion of high-mobility is included
here to distinguish a slurry from concentrated, low-mobility
industrial suspensions of solids and fluid such as gels, greases,
and pastes. The fluid medium of both geophysical and
industrial slurries commonly consists of water but in some
circumstances may consist of a gas or oil. The solids
component of a slurry can have widely varying composition,
size distribution, and concentration. Common geophysical and
industrial slurries can consist of cohesive fine sediment,
inorganic granular debris, rock fragments, coal, animal waste,
sewage sludge, snow, or ice. Some familiar examples of slurries
include freshly mixed concrete, drilling mud, and debris flows.
The volume concentration of solids in a slurry can vary widely.
In general, the finer grained the solids, the lower the
concentration needed to obtain a coherent solids-fluid mixture.
For example, a slurry composed of smectite and water can
become coherent at a solids concentration of as low as 3
percent by volume (Hampton, 1972), whereas a granular
debris flow typically does not become coherent until the solids
concentration reaches about 50 percent or more (e.g., Iverson
and Vallance, 2001).
Distinguishing characteristics of a slurry
A slurry differs from a dilute suspension or solids-laden fluid in
terms of momentum transfer, the pressure in the suspending
fluid, and phase separation of the solid and fluid constituents.
In dilute suspensions or solids-laden fluids, momentum is
transferred by fluid forces, and the solids are a passive
component transported by the fluid (see Gravity-Driven Mass
Flows).
In a slurry, however, significant momentum transfer
occurs through solid-phase interaction, owing to the greater
concentration of particles. Solids are no longer transported
passively, but instead move the suspending fluid in response to
a pressure gradient or gravitational body force.
Nonequilibrium fluid pressure, the total fluid pressure minus
the local hydrostatic fluid pressure, further distinguishes a
slurry from a solids-laden fluid. In a concentrated slurry,
nonequilibrium fluid pressure arises from gravitational settling
of the particles, which transfers stress to the fluid phase. If the
fluid can migrate easily through pore spaces around the solid
particles, the nonequilibrium fluid pressure dissipates readily,
otherwise it remains elevated and helps balance the weight of
the overlying mixture. As long as pore-fluid pressure remains
elevated, particle settling is hindered, and separation of the
solid and fluid phases is limited.
A lack of phase separation over timescales relevant to the
transport process can be used to further distinguish a slurry
from a solids-laden fluid. In a solids-laden fluid, the phases are
not intimately bound, and gravitational settling of particles is
controlled by the size, shape, and density of the particles, by
the density and viscosity of the fluid, and by fluid dynamics
(such as turbulence). Gravitational phase separation in
slurries, however, is related to dissipation of nonequilibrium
fluid pressure (e.g.. Major, 2000; also see Gravity-Driven Mass
Flows).
An approximate estimate of the time necessary for
phase separation to occur in most geophysical slurries is
obtained by examining the ratio h^lD, where /; is the thickness