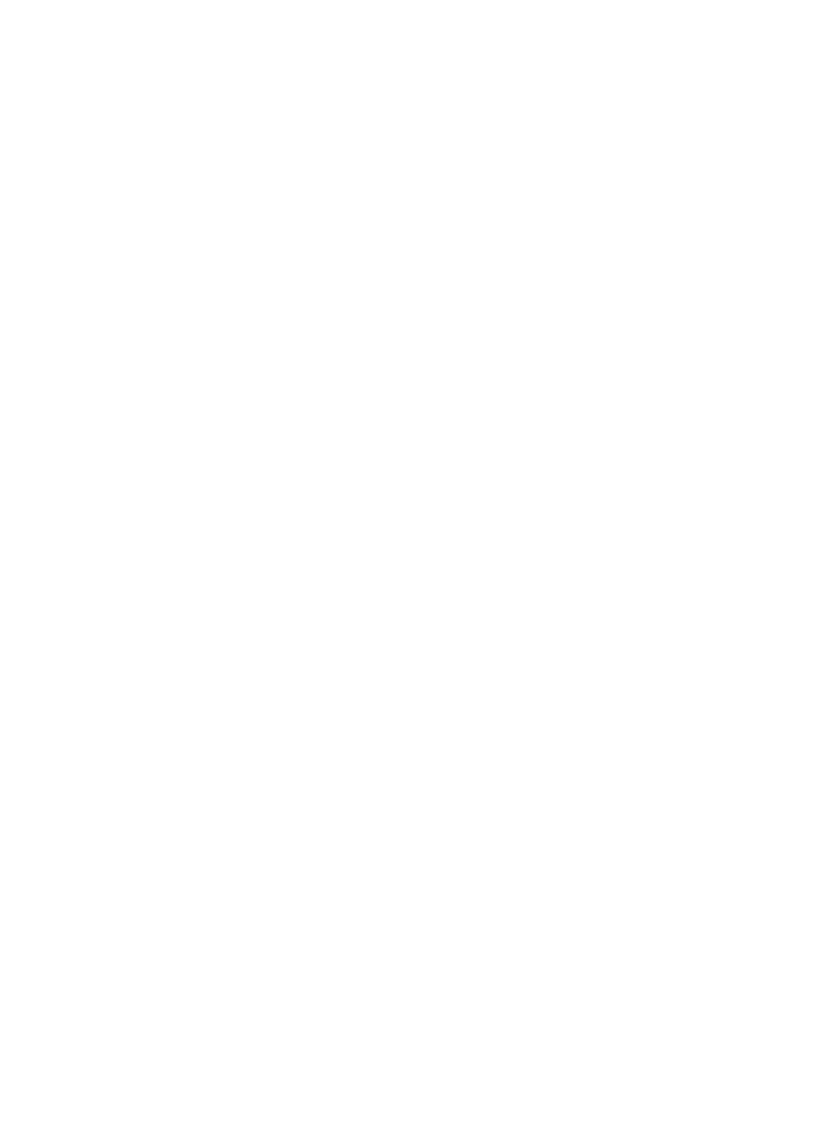
RECHARGEABLE LITHIUM BATTERIES (AMBIENT TEMPERATURE) 34.5
While metallic lithium has the highest specific capacity, it is more reactive than lithium-
aluminum and other alloys. These have been used mainly in small flat or coin cells, as it is
difficult to scale up to larger and spirally wound designs because most lithium alloys are
brittle and cannot be extruded into thin foils. Another approach is the lithium ion battery
which uses a carbon material for the negative electrode. These are attractive from a safety
viewpoint as reactive metallic lithium is not present in the cell. A suitable intercalation
compound is selected for the positive electrode for these cells so that a high cell voltage is
obtained. The cell operates by the lithium ions shuttling back and forth between the elec-
trodes during the discharge-charge cycle. No metallic lithium is plated during the charge and
no metallic lithium is present in the cell.
Lithium Metal. The search for high-energy-density batteries has inevitably led to the use
of lithium, as the electrochemical characteristics of this metal are unique. A number of
batteries, both primary and rechargeable, using a lithium anode in conjunction with inter-
calation cathodes, were developed which had attractive energy densities, excellent storage
characteristics, and, for rechargeable cells, a reasonable cycle life. Commercial success has
eluded all but the primary batteries due to persistent safety problems.
The difficulties associated with the use of metallic lithium stem from its reactivity with
the electrolyte and the changes that occur after repetitive charge-discharge cycling. When
lithium is electroplated, during recharge, onto a metallic lithium electrode, it forms a mossy
and in some cases a dendritic deposit with a larger surface area than the original metal.
While the thermal stability of lithium metal foil in many organic electrolytes is good, with
minimal exothermic reaction occurring up to temperatures near the melting point of lithium
(181
⬚C), after cycling the surface area of the lithium increases significantly with a corre-
sponding increase in the reactivity. This lowers the thermal stability of the system, with the
result that cells become increasingly sensitive to abuse as they are cycled.
Another contributing effect is the inability of attaining 100% lithium cycling efficiency.
This happens because lithium is not thermodynamically stable in the organic electrolytes and
the surface of the lithium is covered with a film of the reaction products between the lithium
and the electrolyte. Every time the lithium is stripped and replated during discharge and
charge, a new lithium surface is exposed and then passivated with a new film, consuming
lithium. Because of the mossy deposit, some lithium becomes electrochemically unreactive
on repeated cycling. In order to obtain a reasonable cycle life, a three- to fivefold excess of
lithium is required.
The failure to control the surface area of the lithium anode remains a problem, limiting
the commercialization of lithium anode cells with liquid organic electrolytes. Given this
problem, an alternate solution is to use an electrolyte, such as a solid polymer electrolyte,
which is less reactive with lithium. This is covered in Sec. 34.2.3.
Carbon Materials. In the lithium-ion cell, carbon materials, which can reversibly accept
and donate significant amounts of lithium (Li:C
⫽ 1:6) without affecting their mechanical
and electrical properties, can be used for the anode instead of metallic lithium. Carbon
material is used as an anode in lithium-ion cells since the chemical potential of lithiated
carbon material is close to that of metallic lithium, as shown in Fig. 34.2. Thus an electro-
chemical cell made with a lithiated carbon material will have almost the same open-circuit
voltage as one made with metallic lithium. In practice, the lithium-ion cell is manufactured
in a fully discharged state. Instead of using lithiated carbon material, which is air-sensitive,
the anode is made with carbon and lithiation is carried out by subsequent formation of the
cell.
The specific energy or capacity of the lithium-ion system depends largely on the type of
carbon materials used, the lithium intercalation efficiency, and the irreversible capacity loss
associated with the first charge process. Table 34.3 lists the properties of some of these
carbon materials. Coke-type carbon, having physical properties such as ash content
⬍0.1%,
surface area
⬍10 m
2
/g, true density ⬍2.15 g/cm
3
, and interlayer spacing ⬎3.45 A
˚
, were
used in first-generation lithium-ion system. These types of carbon materials can provide about