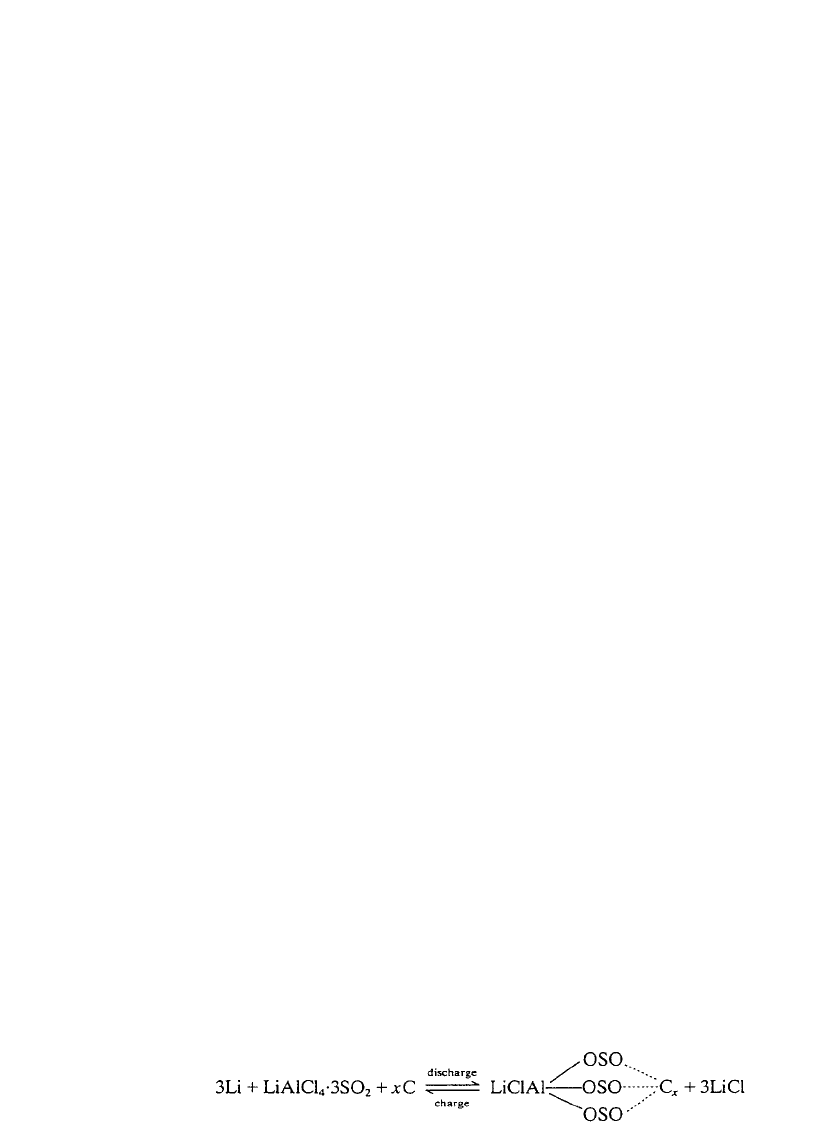
RECHARGEABLE LITHIUM BATTERIES (AMBIENT TEMPERATURE) 34.21
One of the major problems of lithium polymer electrolyte systems is the development of
high interfacial resistance at the lithium/ polymer electrolyte interphase.
20
This resistance
grows with time and could be as high as 10 k
⍀ cm
⫺
2
.
21
This resistance layer is due to the
reactions of lithium with water, other impurities, and the salt anions. Similar to nonaqueous
electrolytes, the solid electrolyte interphase (SEI) also exists in the lithium/ polymer electro-
lyte systems.
22
In this case, the solid electrolyte interface (SEI) consists of the inorganic
reduction products of the polymer electrolyte and its impurities.
The capacity of a lithium polymer electrolyte battery decreases gradually on cycling. This
capacity loss is most likely related to degradation at the electrode interfaces due to repeated
plating-stripping cycles at the lithium metal anode and the repeated intercalation-
deintercalation cycles at the composite cathode. The cycling performance of lithium polymer
electrolyte cells can be improved by reducing the lithium passivation phenomena and by
improving the cathode morphology and homogeneity (particle size).
The energy density of the SPE batteries is projected to be close to that of the liquid-
electrolyte lithium rechargeable batteries. The thin cell design, however, usually requires a
larger percentage of materials of construction and nonreactive components, which tend to
lower the energy density. Larger electrode areas are also required for a given cell capacity
than with conventional battery design. The thin separator and larger electrode area could
adversely affect the cycle life, safety, and reliability of the battery by increasing the chance
for internal short circuits, lithium dendrite penetration, and other deleterious effects.
Another potential advantage of the solid polymer battery is that the design lends itself to
manufacture by automatic processes and the capability to be easily fabricated in a variety
of shapes and forms. Very thin batteries for cell phones, PDAs and similar applications can
be manufactured. At the other extreme, large thin plates can be manufactured and assembled
in multiplate prismatic or bipolar batteries.
Solid polymer batteries using lithium metal have not yet been introduced in the market-
place, and most of the performance characteristics are based on laboratory prototypes. The
technology offers several potential advantages compared to the other rechargeable lithium
batteries, including high energy density, low self-discharge rate, improved safety, and thin
cell design. The success of this approach will depend on the ability to achieve these advan-
tages, first in small cells and batteries and then to be able to scale up the technology to the
larger-size cells and multicell batteries required, for example, for electric vehicles. Lithium
ion batteries using polymer electrolytes have been introduced to the market in 2000.
Inorganic Electrolyte Batteries. The rechargeable lithium batteries with SO
2
-based inor-
ganic electrolytes are attractive because they can operate at high charge and discharge rates
due to the high ionic conductivity of the electrolyte. Other advantages are a high energy
density, a low self-discharge rate, the ability to accept limited overcharge and overdischarge,
and a wide electrochemical voltage window (5.0 V versus lithium).
Their drawback is the toxicity of the electrolyte and concern with safety problems. High-
capacity fade and relatively low capacity at low temperatures are other disadvantages.
Several types of batteries have been investigated using the SO
2
-based electrolyte cells
(with LiAlCl
4
as a solute) and metallic lithium for the negative electrode. These use either
carbon
11
or CuCl
2
26
for the positive electrode material.
With a high-surface-area carbon black such as Ketjen black, the SO
2
-based LiAlCl
4
elec-
trolyte forms a complex which is believed to take part in the discharge-charge process as