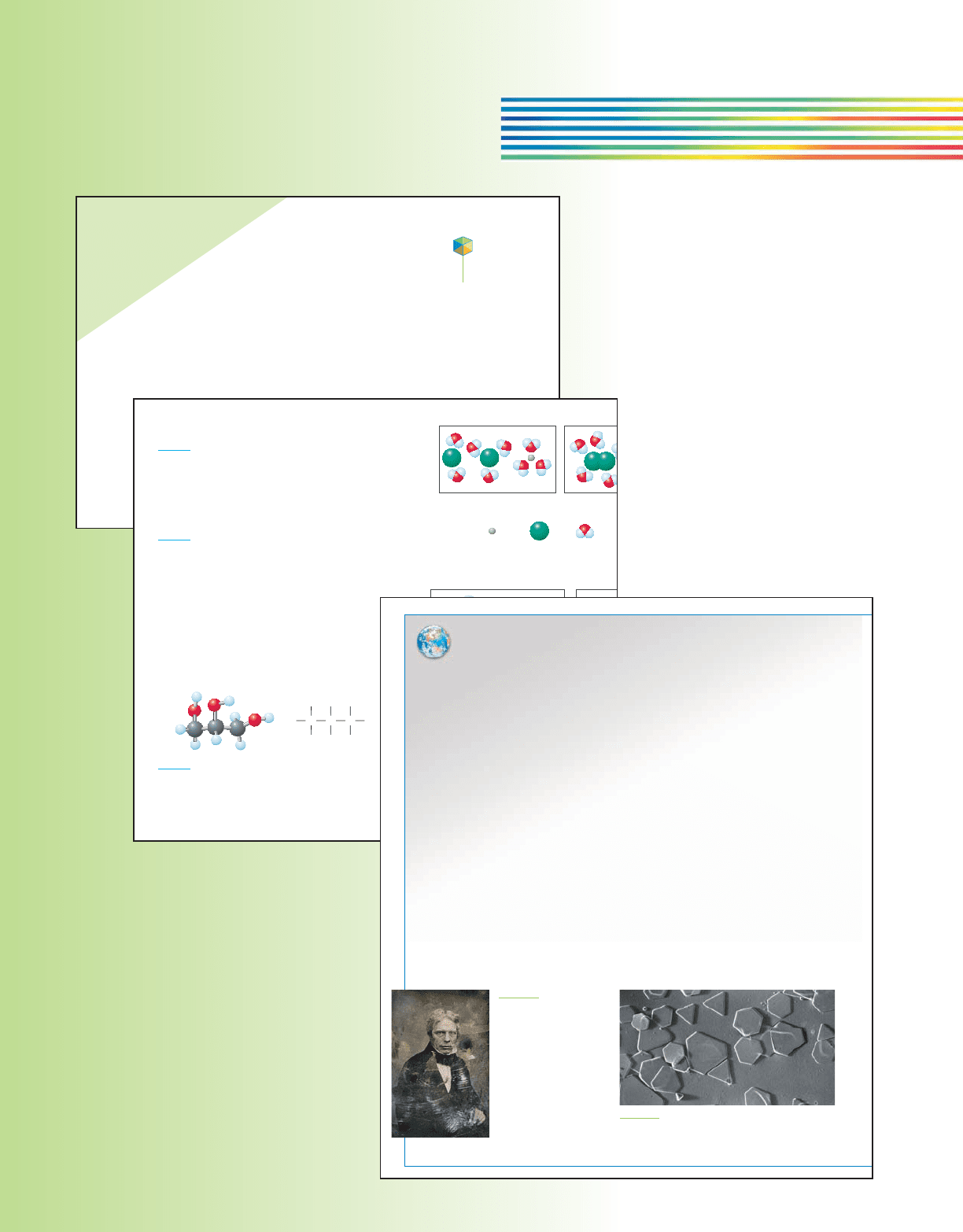
vi
Engage students through
integrated applications
A space shuttle launch is an awe-inspiring
demonstration of the ability of energy to trans-
port humans and material away from Earth’s surface
with an eye toward planetary exploration. The energy to
launch the craft and its crew comes from the explosive vio-
lence of chemical reactions. When these reactions are used in a
carefully controlled way, they can lift the massive shuttle (which
weighs in at a robust 2,000,000 kg), the booster rockets, and the fuel tank
and propel the ship into orbit hundreds of miles above Earth in a very brief
10-minute ride.
Two chemical reactions, indicated in Figure 5.1, power the launch of the space
shuttle. The combustion of hydrogen gas (the combination of hydrogen and oxygen
to form water) takes place in the main engines. At the same time, the solid rocket
boosters attached to the sides of the shuttle release a host of products resulting from
the oxidation of aluminum by ammonium perchlorate, though we show only the
primary equation here.
Main engines: 2H
2
(g) + O
2
(g) →2H
2
O(g)
Boosters: 10Al(s) + 6NH
4
ClO
4
(s) → 5Al
2
O
3
(s) + 6HCl(g) + 3N
2
(g) + 9H
2
O(g)
As the shuttle sits on the launch pad, it is hard to believe that the en-
ergy that will launch it with such a spectacular display of chemical mus-
cle is quietly present within the main fuel tank and the solid fuel of the
booster rockets. Chemicals can store huge amounts of energy in this
Application
C
HEMICAL ENCOUNTERS:
Setting the Stage with
the Space Shuttle
Interwoven throughout the chapter,
chemistry concepts are explained
through the use of real-world examples.
Application icons throughout the text
show at a glance where chemical
concepts are applied.
Applications are also found
in
How Do We Know? and
NanoWorld / MacroWorld
features, chapter openers,
photos, and end-of-chapter
exercises. Students will see how
chemistry connects to their lives,
their future careers, and their
world.
4. Diagram, using circles for atoms, a crystal of KCl versus the
same crystal of KCl dissolved in water.
Chemical Applications and Practices
5. Earth’s oceans contain tons of dissolved sodium chloride.Yet,
when a ship develops an oil leak, almost none of the oil dis-
solves in the ocean. Explain this phenomenon.
6. When an ion dissolves, it is surrounded by a hydration
sphere. If water molecules surrounded the ion so that the hy-
drogen portion of the water was closer to the ion, would the
ion most likely be a cation or an anion?
7. Pure water does not conduct an electric current. However,
aqueous solutions of some compounds do form solutions
that conduct electricity. Explain why the presence of some
solutes converts nonconducting water into a conducting
solution.
8. Glycerin can be produced as a by-product in soap making.
The compound dissolves so easily in water that it absorbs
water from the air. This latter characteristic is why glycerin
is often found in many skin lotions. As glycerin absorbs
the water, the skin can be kept moist. Glycerin’s structure is
shown below. What aspects of glycerin’s structure contribute
most to its ease of dissolving in water?
9. A conductivity-testing apparatus, such as the one shown in
this chapter, possesses a light bulb whose brightness is related
to how much current is flowing through it (and also through
the solution.) A small, but measurable, amount of current
must be present before the bulb becomes visibly brighter.
What effect would this characteristic of the apparatus have
12. Which of the following would best represen
water?
CHH
H
OH
C
H
OH
C
H
OH
Gl
cerin
(a) (b)
(c) (d)
Mg
2+
H
2
OCl
–
(a) (b)
(c) (d)
“Smile, you’re on candid camera!” Everyone hopes never
to be ambushed with those words. In the nineteenth cen-
tury, when photography was in its infancy, candid pho-
tographs were not possible. In order to create a visual
memory of an event, you had to visit the photographer
and sit for a picture.“Sitting” meant holding a pose for
up to 30 seconds. Any movement would show as a blur
in the final picture. However, the idea that an inexpen-
sive, yet realistic, picture could be created in such a short
time brought people to the photographer’s studio in
droves.
Scientists in the early 1800s were intrigued by the
idea of painting portraits without the artist’s pen. In the
summer of 1827, Joseph Nicéphore Niépce, using mater-
ial that hardened on exposure to light exposed film for
eight hours in order to get a picture. Because no one
wanted to sit still that long, most of his pictures were
landscapes. In 1839, however, two other photographic
processes were developed, the daguerreotype (perfected
by Louis Daguerre) and the calotype (Henry Fox Talbot).
Although these techniques allowed pictures to be created
with much shorter exposures, they still produced a
black-and-white image (see Figure 9.30). This didn’t
matter much to the general public, and photographic
portrait studios seemed to pop up on every corner across
the globe. Despite some marginal success in producing
faint color images during the 1850s and 1890s, the ad-
vent of the color photograph as an inexpensive, repro-
ducible and stable process didn’t occur until the 1920s.
Researchers at Kodak Research Laboratories in 1935
finally hit upon the process that would bring the color
photograph to the world. Kodachrome®, their color
photography process, introduces color to a picture
by separating the three primary colors into specific
emulsion layers. How does it work? The emulsion layers
contain a compound that reacts when light strikes it. A
quick look at the chemistry behind the black-and-white
photograph will give us some insight.
In black-and-white photography, a piece of transpar-
ent plastic is coated with silver bromide crystals (see Fig-
ure 9.31) and a sensitizer. The plastic sheet is then placed
in a camera, and when the shutter is opened, the silver
bromide absorbs photons of light. In the presence of the
sensitizer, the silver cation is reduced by the sensitizer
(gains an electron) and becomes silver metal:
Ag
+
(s) + sensitizer n Ag°(s) + sensitizer
+
Then the film is wound back into its container and
sent to be developed. The technician rinses the plastic
film to remove unreacted silver bromide. The silver
metal remains in place on the plastic. The result is an in-
verted image called a negative. Next the technician shines
light through the negative onto another silver bromide/
sensitizer-coated support (typically paper this time) and
rinses off the unreacted silver bromide. Violà! We have a
positive image called a photograph.
Why is the film coated with silver bromide instead of
silver chloride? We can answer this by examining the
wavelength of light that is absorbed; see Figure 9.32.Com-
parison of the two shows that silver bromide absorbs a
large amount of the visible light that enters our camera.
To get the best picture, we want to absorb as much light
as we can.
NanoWorld / MacroWorld
Big effects of the very small:
Color photography and the tricolor process
FIGURE 9.30
A daguerreotype of Michael Faraday
(see Chapter 19) taken sometime
between 1844 and 1860 at Mathew
Brady’s studio in New York City.
Because the process required the
subject to remain completely
motionless for up to 10 seconds,
most daguerreotypes were taken
in well-lit studios. Even though
the early daguerreotypes cost one
month’s wages for the average
person, they were much cheaper
than a painted portrait. This made
them an instant success.
FIGURE 9.31
A magnified image of silver bromide crystals used in the photographic
industry. Note that the crystals are mostly hexagonal in shape and flat
so that more surface area is exposed to incoming photons.