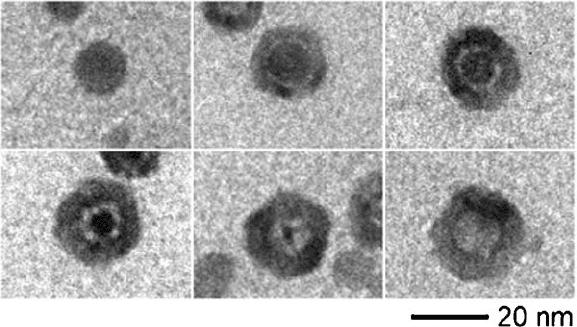
etc. There are a copious number of applications for nanostructural oxide s such as
high-density magnetic storage, heterogeneous catalysis, gas sensors, electrolytes for
lithium batteries, and fuel cells. Sun and coworkers recently describ ed a polyol
[146]
route for the synthesis of hexane-suspended Fe
3
O
4
(magnetite) nanoclusters through
the reaction between Fe(acac)
3
, oleylamine, oleic acid, and 1,2-hexadecanediol at
ca. 200
C within phenyl ethe r.
[147]
This one-pot synthesis results in the thermal
replacement of the acetylacetonate ligands (recall Chapter 4 – CVD precursors)
from the iron center, followed by oxide formation from reaction with the alcohol.
The oleylamine and oleic acid condense in situ to form a long-chain inverse micelle
that is capable of suspending the nanoclusters in nonpolar media. For purification,
one simply adds an excess of polar solvent (methanol or ethanol) to precipitate
the nanostructures. Following centrifugation, the nanocl usters are re-suspended in
hexane, with this precipitation/resuspension procedure repeated three to four times
to afford monodispersed, chemically pure Fe
3
O
4
nanoclusters. Another analogous
procedure uses oleic acid/trimethylamine N-oxide in trioctylamine (b.p. 365
C) to
decompose Fe(CO)
5
to yield monodispersed g-Fe
2
O
3
nanoclusters.
[148]
Interest-
ingly, shape control of nanoparticles may be afforded by altering the nature of the
solvent or injection timing of surfactants.
[149]
Another more recent strategy that we developed is the synthesis of metal oxide
nanoparticles from the simple reaction of dendritic-stabilized basic metalate salts
with CO
2
(Figure 6.51).
[150]
This strategy should work for any metalate salt that is
typically formed from the reaction of the metal hydroxide with a strong base (Eq. 11,
for sodium hafnate). Metal/nonmetal oxides may also be synthesized using a sol-gel
technique, analogous to that described in Chapter 4 for thin film growth. Fo r
Figure 6.50. Hollow nanoshell formation via the Kirkendall Effect. Shown is the evolution of the hollow
nanoshell after reaction times of (left–right): 0 s, 10 s, 20 s, 1 min, 2 min, 30 min. An interesting feature is
the formation of “bridges” that connect the core to the sulfide shell, facilitating the fast outward migration
of Co. Reproduced with permission from Yin, Y.; Rioux, R. M.; Erdonmez, C. K.; Hughes, S.; Somorjai,
G. A.; Alivisatos, A. P. Science 2004, 304, 711. Copyright 2004 AAAS.
6.3. Nanoscale Building Blocks and Applications 517