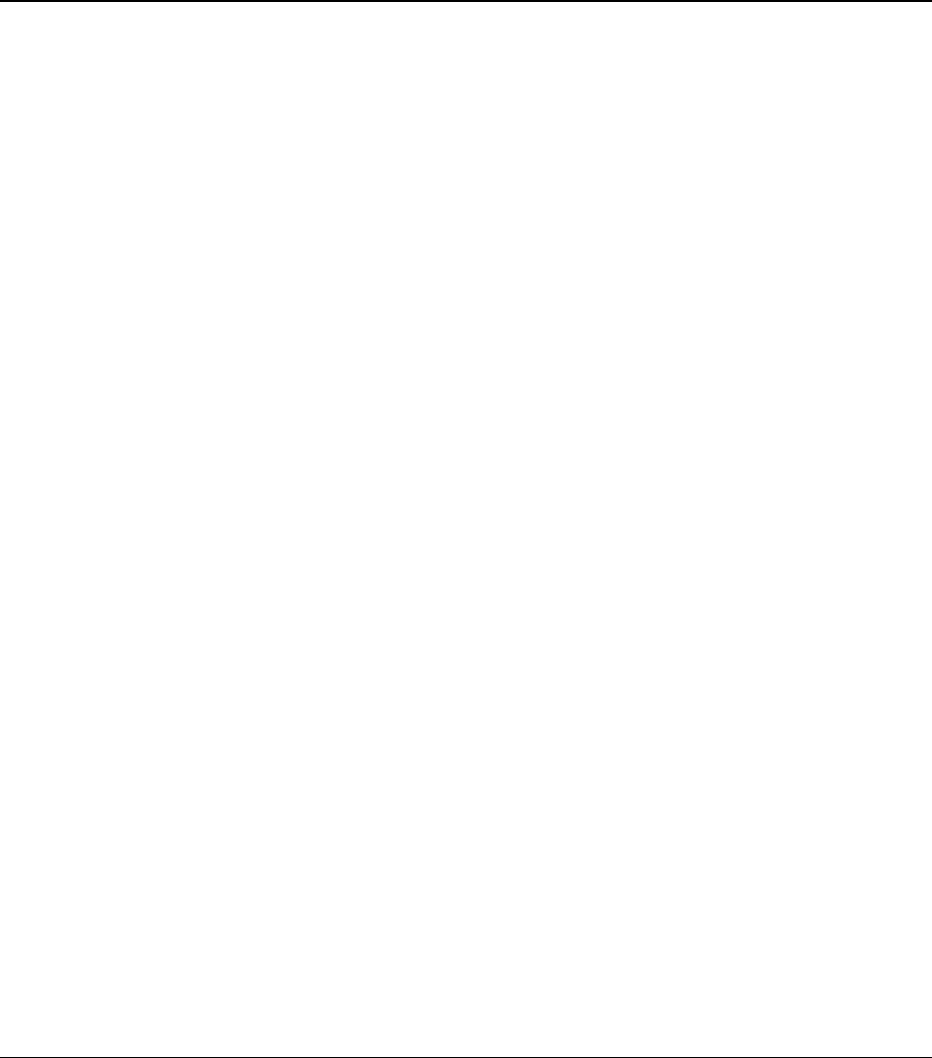
may not be typical. There was very little taphonomic
control in this study. At each interval the distance, as
measured by number of character changes along the
inferred lineage between terminal taxa, was averaged
over all pair wise comparisons. This gave an average
morphological distance for successive time periods in
the clade’s history. The values for successive time
periods could then be compared to each other and,
in turn, compared to the number of cladogenetic
events within each time period (which provided a
measurement of taxic diversity). In this case the
taxic diversity tracked morphological diversity except
for the early stages where taxic diversity slightly
exceeded morphological diversity.
Some other studies have not considered the phylo-
genetic relationships within clades (although mono-
phyly of the entire clade has been accepted). Figure 11
shows the results of such a study of the radiation of
members of the echinoderm subphylum Blastozoa
(see Fossil Invertebrates: Echinoderms (Other Than
Echinoids)). In this study, one species each from
147 genera, ranging in time from the Lower Cam-
brian to Permian, were examined for 65 morpho-
logical characters. Ten stratigraphic intervals were
chosen and within each interval pair wise compari-
sons between characters coded for each of the genera
were made and averaged. The phylogeny was not
considered here. One common belief is that morpho-
logical diversity is disproportionately high compared
to taxic diversity at the beginning of a radiation
and that such a relationship is reversed later on. The
blastozoan study appears to confirm this since the
morphological diversity was far greater than
the taxic diversity in the mid-Cambrian to Lower
Ordovician; that is, in the early stages of clade
history. The reverse relationship was true in the
Lower Carboniferous.
The Cambrian explosion must be the most spec-
tacular of all radiations that the planet has witnessed.
Yet it remains one of the most enigmatic. Within
15 million years of the beginning of the Cambrian
period, where tiny shelly faunas are first detected, a
wide variety of skeletonized animals appeared, al-
ready well diversified and exquisitely adapted. Today
they are classified amongst the major invertebrate
phyla. Molecular evidence, alongside phylogenetic
studies on the first appearing fossils all point to there
being a long Precambrian history to many lineages.
But exactly what form this history took is very uncer-
tain. Steven Jay Gould, in his book Wonderful Life,
– suggested that when skeletonized animals first
appeared in abundance in the Cambrian there was a
remarkably diverse array of body forms and that
many of these were weeded out by extinctions leading
to differential survival of a few body forms that
eventually gave rise to our modern fauna. In other
words, morphological disparity was greatest in the
early phase of the animal lineage history and gradually
became canalized. On a broad scale this theory echoed
the radiation pattern shown by the blastozoan echino-
derms mentioned above. Gould’s thesis was grounded
in a large part on the arthropods, and especially the
crustacean-like animals, conspicuous in the famous
Burgess Shale Lagersta
¨
tten, but was largely anecdotal,
with little phylogenetic evidence or any precise meas-
ures of morphological disparity applied. More
detailed studies comparing levels of disparity in Cam-
brian and Recent arthropods (see Fossil Invertebrates:
Arthropods) showed that there was very little differ-
ence between then and now. More specifically detailed
studies of the early evolution of the Crustacea have
shown that morphological disparity grew in concert
with taxic diversity (Figure 12).
The study of the pattern of radiations in the fossil
record has implications for our understanding of post-
extinction recovery and potentially for our efforts to
put conservation efforts in place. If there are general
patterns that emerge that can be associated with
Figure 12 Morphological disparity of crustaceans tracked through time against a phylogeny. In this study one exemplar taxon from
each of the crustacean orders or suborders was coded for 135 characters. (A) A cladistic analysis plotted against stratigraphy resulted
in the consensus tree shown here. The actual stratigraphic ranges are shown in black and those which must be assumed because
of the shape of the tree in grey (ghost ranges). Notice that most of the species of modern crustacea did not appear until late Palaeozoic.
(B) Plots showing disparity of the clades present through three time slices. The disparity is measured by making pair wise
comparisons between the coded characters for each of the taxa present in any one time interval (the ghost range taxa were coded
according to reconstructed ancestral states). Some differential weights were applied according to the particular type of character and
in this it differed from the Blastozoan study (Figure 11). A principal coordinate analysis was then carried out to illustrate the difference
in morphospace between the taxa. In the diagrams the first three principal coordinates are shown, the third coordinate being at right
angles to the paper (filled symbols positive and open symbols negative). The black symbols represent taxa with a range extending
through the stage illustrated, red symbols represent taxa implied by ghost ranges and the green symbols are taxa extinct at stage
illustrated. This diagram shows that both morphological disparity and taxic diversity increased from the Cambrian to Upper Devonian
at which time a new area of morphospace (bottom left) was occupied. Reproduced from Wills MA (1998) Crustacean disparity through
the Phanerozoic: comparing morphological and stratigraphic data.
Biological Journal of the Linnean Society of London 65: 455–500.
278 BIOLOGICAL RADIATIONS AND SPECIATION