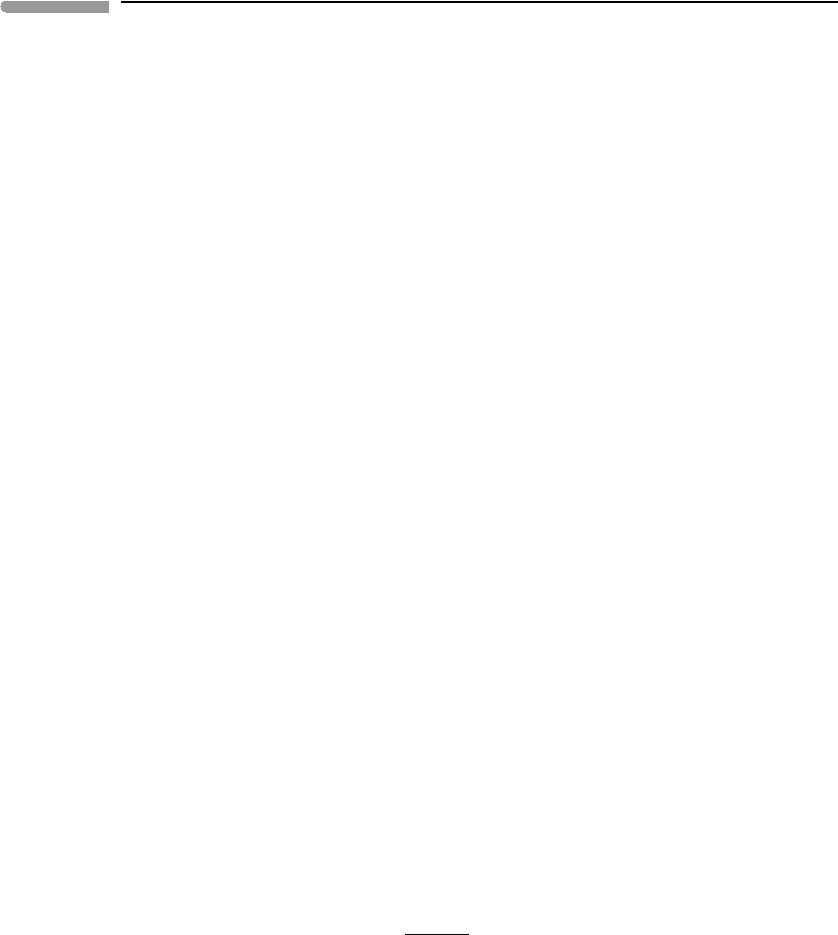
560 Dilute solutions
ferric iron remains very low and constant, at close to 10
−12
molal, until magnetite starts
precipitating, where it decreases further with decreasing oxygen fugacity. Above ∼10
−50
bar oxygen fugacity the concentration of total dissolved ferrous iron becomes negligibly
small and iron solubility is determined by the solubility of ferric species.
The total concentration of dissolved iron in Archaean seawater in equilibrium with an
anoxic atmosphere in which f (O
2
) was less than about 10
−50
bar may have been several
orders of magnitude higher than the solubility of iron in today’s shallow oceans. Dissolved
iron in Archaean oceans would have been overwhelmingly present as ferrous species, that
could precipitate to solid ferric phases upon oxidation. Iron solubility remains essentially
unchanged over an f (O
2
) range of ∼40 orders of magnitude, from 10
−40
bar to its present-
day value. Deposition of banded iron formations by oxidation of dissolved ferrous iron
must have taken place under oxidation conditions (f (O
2
) <∼10
−50
bar) that are not even
remotely comparable to those that are needed for aerobic metabolism (Chapter 14).
The equations show that iron solubility in water is a function not only of oxygen fugacity
but also of pH, and the question arises, could the deposition of banded iron formations
primarily reflect a change in oceanic pH, rather than in oxygen fugacity? In Exercise 11.6
you can explore this and see why, although possible, this is a very unlikely explanation –
BIF deposition must be the response to oxidation of the Earth’s surficial environments.
Figure 11.7 shows a subtle detail that is not evident from the schematic phase diagrams
that we constructed in Worked Example 6.7. This is the fact that formation of magnetite
iron formations requires more concentrated iron solutions than formation of hematite iron
formations. For a total iron concentration lower than that at the magnetite–hematite transi-
tion (∼6×10
−8
molar in this simplified model) magnetite iron formations cannot form at
any oxygen fugacity, but hematite iron formations would form by oxidation.
In Worked Example 6.7 we saw that magnetite iron formations are sometimes associated
with siderite, and that some Precambrian BIFS are in fact composed chiefly of siderite. In
order to study the conditions that lead to the formation of siderite iron formations we begin
by writing the following magnetite–siderite equilibrium:
2Fe
3
O
4
+6CO
2
6FeCO
3
+O
2
. (11.119)
The oxygen fugacity at which siderite replaces magnetite as the iron solubility-limiting
phase depends on the fugacity of carbon dioxide. Using the pure gases at the temperature of
interest and 1 bar as the standard states for O
2
and CO
2
we write the equilibrium condition
for (11.119) as follows:
f
O
2
f
CO
2
6
=K
mt−sd
(11.120)
with K
mt−sd
= exp(−
r
G
0
/RT ) = 2.887 ×10
−70
at 298 K (data from Wagman et al.,
1982). As an example, let us assume that CO
2
fugacity in the Archaean atmosphere was
0.02 bar. A value considerably higher than today’s is suggested by the fact that Archaean
glaciations appear to be non-existent, despite the fact that the early Sun was fainter than
today’s (Sagan & Mullen, 1972; Kasting, 1987; Gilliland, 1989). From this CO
2
fugacity
we calculate an oxygen fugacity at the magnetite–siderite transition of ∼1.9 × 10
−80
bar,
i.e. well below the hematite phase transition. An important question is whether CO
2
is stable
at this low oxygen fugacity, relative to, for example, CO. The answer, which you are asked
to prove in Exercise 11.7, is yes.