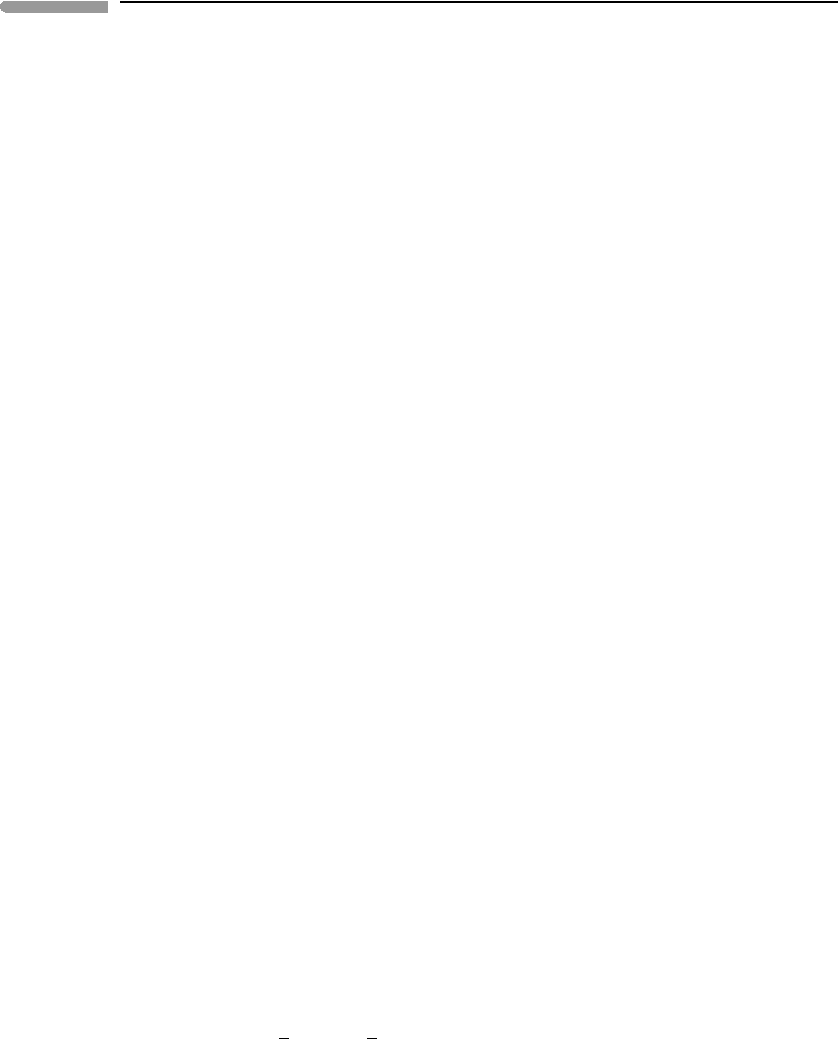
555 11.5 Speciation in ionic solutions
magnitude higher than the oxygen fugacity along the hematite–magnetite buffer at room
temperature (Fig. 9.3). We can expect the solubility-limiting phase to be either ferric oxide
or ferric hydroxide. The Gibbs free energy change for the reaction 2 Fe(OH)
3
→ Fe
2
O
3
+
3H
2
Oat298Kis∼−30 kJ mol
−1
, which means that hematite is the stable phase.
The phase that usually precipitates from seawater is not hematite, however, but rather
an amorphous solid or a hydrated crystalline ferric oxide with a composition intermedi-
ate between those of hematite and ferric hydroxide. Over time the precipitate dehydrates
and becomes hematite, and this may have been the mechanism by which Precambrian
hematite iron formations formed. This is a kinetic problem, that we will not address here
(see Chapter 12). We will use hematite in our calculations, in order to be consistent with
Worked Example 6.7. Because the metastable phase that actually forms has higher Gibbs
free energy than hematite, the results of our calculations are an absolute minimum of
solubility.
In these calculations we will only consider dissolved iron species that exist in an aqueous
solution with no other solutes, except carbonate anions. The effect of this simplification is
to underestimate the solubility of iron relative to the actual solubility in seawater, in which
formation of other Fe-bearing ionic species is possible. We will also assume that the activity
of dissolved iron species is ideal (i.e. a = m). Our estimated total iron contents in solution
will be about two orders of magnitude lower than the analytical concentrations of iron in
present day seawater (∼10
−10
molal in oxygen-rich near-surface water). The qualitative
behavior with changes in oxygen fugacity and pH that we will uncover are, however, robust,
and will illuminate the significance of banded iron formations (see also Worked Example
6.7). A rigorous discussion of iron solubility in seawater is given, for example, by Millero
et al.(1995) and Liu and Millero (2002).
We can think of dissolution of Fe ionic species in water as occurring in one of two ways.
The one that is perhaps more intuitively appealing is to think of iron hydroxides as weak
bases. Just as dissolved CO
2
, which is a weak acid, undergoes successive ionization reactions
(Worked Example 11.3), so a molecular aqueous species such Fe(OH)
3aq
can be thought
to dissociate in steps to Fe(OH)
2
+
, FeOH
2+
and finally Fe
3+
. All of these stoichiometric
species are known to exist in ferric iron solutions. Alternatively, we may imagine that Fe
3+
exists in solution as free ions that associate electrostatically with OH
−
groups to form
complex ions with the same stoichiometries as the molecular species that would form by
dissociation. The difference is that whereas in the former case the intermediate ionic species,
as well as neutral Fe(OH)
3
, are taken to be covalently bonded molecules, in the latter case the
ionic species are complexes in which Fe
3+
and OH
−
ions are attached electrostatically. The
more accurate physical picture is the latter one, but from a macroscopic thermodynamic
point of view the two approaches are equivalent. I will therefore use the “weak base”
model, because of its intuitive appeal. According to this model dissolution of hematite in
water proceeds as follows:
1
2
Fe
2
O
3
+
3
2
H
2
O Fe
(
OH
)
3aq
(III,hm)
Fe
(
OH
)
3 aq
Fe
(
OH
)
+
2 aq
+OH
−
aq
(III,1)
Fe
(
OH
)
+
2 aq
FeOH
2+
aq
+OH
−
aq
(III,2)
FeOH
2+
aq
Fe
3+
aq
+OH
−
aq
(III,3)
(11.107)