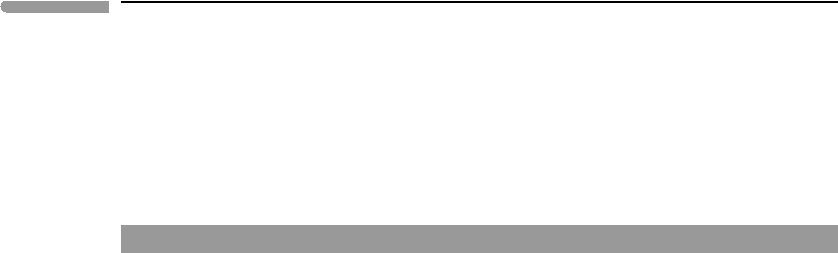
344 Phase equilibrium and phase diagrams
“eutectic” gas shifts in the direction of the more volatile substance. These may have been
important factors in the condensation of solids from nebular gas during formation of the
Solar System. For example, a solid of relatively low volatility such as B in the bottom panel
of Fig. 6.22 may have been prevented from condensing by the presence of more volatile
components in the nebular gas, such as A in the figure, until the nebular gas attained a
pressure considerably higher than what would have been needed to condense the pure solid.
Worked Example 6.10 Cryolavas in Triton
One of the biggest surprises to emerge from the flyby of the Neptune system by Voyager 2
in 1989 was the discovery that its large satellite Triton has a tenuous atmosphere and shows
signs of current, or at least recent, geological activity. There are stunning pictures of what
are almost certainly volcanic calderas and associated lava flows. Icy worlds such as Triton
undergo volcanic processes (and, necessarily, plutonic ones) that may resemble those in
terrestrial planets in many ways except one: the composition of the magmatic liquids. The
temperatures and compositions of icy crusts do not allow the existence of silicate liquids,
but melts composed of mixtures of C–H–O–N species, known as cryolavas if they are
erupted, are certainly possible. Melting in the binary system CH
4
–CO is a simple example
of cryomagmatism that may be applicable to Triton (Fig. 6.23). Actual icy magmas are no
doubt more complex and varied than this. Using this phase diagram as an introduction to
cryomagmatism is akin to introducing the study of basaltic magmatism with the system
diopside–anorthite – an informative simplification.
The melting points of pure CH
4
and CO at 1 bar are 90.6 K and 68.1 K, respectively.
These values would of course be different at the higher pressures at which the cryomagmas
would form in Triton’s interior, but we will ignore that. The enthalpies of melting are 0.93
and 0.84 kJ mol
−1
for CH
4
and CO, respectively. Assuming that the two species crystallize
as pure immiscible solids and that they mix ideally in the liquid phase we use the system of
equations (6.70) and (6.71) to calculate the phase diagram in Fig. 6.23 (see Software Box
6.1). The system melts at a eutectic temperature of ∼51 K, and the eutectic melt consists of
approximately 60 mol% carbon monoxide and 40 mol% methane.
Magmas on Earth seldom reach the surface at temperatures significantly above their
liquidus (Chapter 10). If this is also the case in icy satellites then one could expect cryolavas
to have temperature–composition combinations along one of the two liquidus curves on
Fig. 6.23, and perhaps to be saturated with phenocrysts of either methane ice or carbon
monoxide ice, depending on the bulk composition. Say that a cryolava of composition L
(Fig. 6.23) is erupted at a temperature of ∼65 K on Triton’s surface, where the ambient
temperature is about 38 K. The saturation vapor pressures of liquid CH
4
and CO at 65 K
are about 1 and 94 mbar, respectively, which are orders of magnitude higher than Triton’s
atmospheric pressures (tens of µbar, see Worked Example 6.11). The lava will therefore
boil upon eruption, but boiling will increase its cooling rate, so that a quenched crust
(microcrystalline? glassy?) is likely to form on the surface of the lava flow. Say that this
crust cools instantaneously to the ambient 38 K. The saturation vapor pressures of solid CH
4
and CO at 38 K are, respectively, ∼0.006 and 2.6 µbar, i.e., below atmospheric pressure.
The solid crust is therefore stable against sublimation in Triton’s atmosphere (but see also
next Example). If the lava flow is thick enough, or the liquid collects in a lava lake, cooling
and crystallization of the interior of the flow may progress slowly enough that the liquid
composition will move down the liquidus curve, crystallizing methane ice, as shown by the
arrow in Fig. 6.23. An interesting question is what happens to these crystals – do they float
or sink? This depends on how the density of methane–carbon monoxide liquids varies with