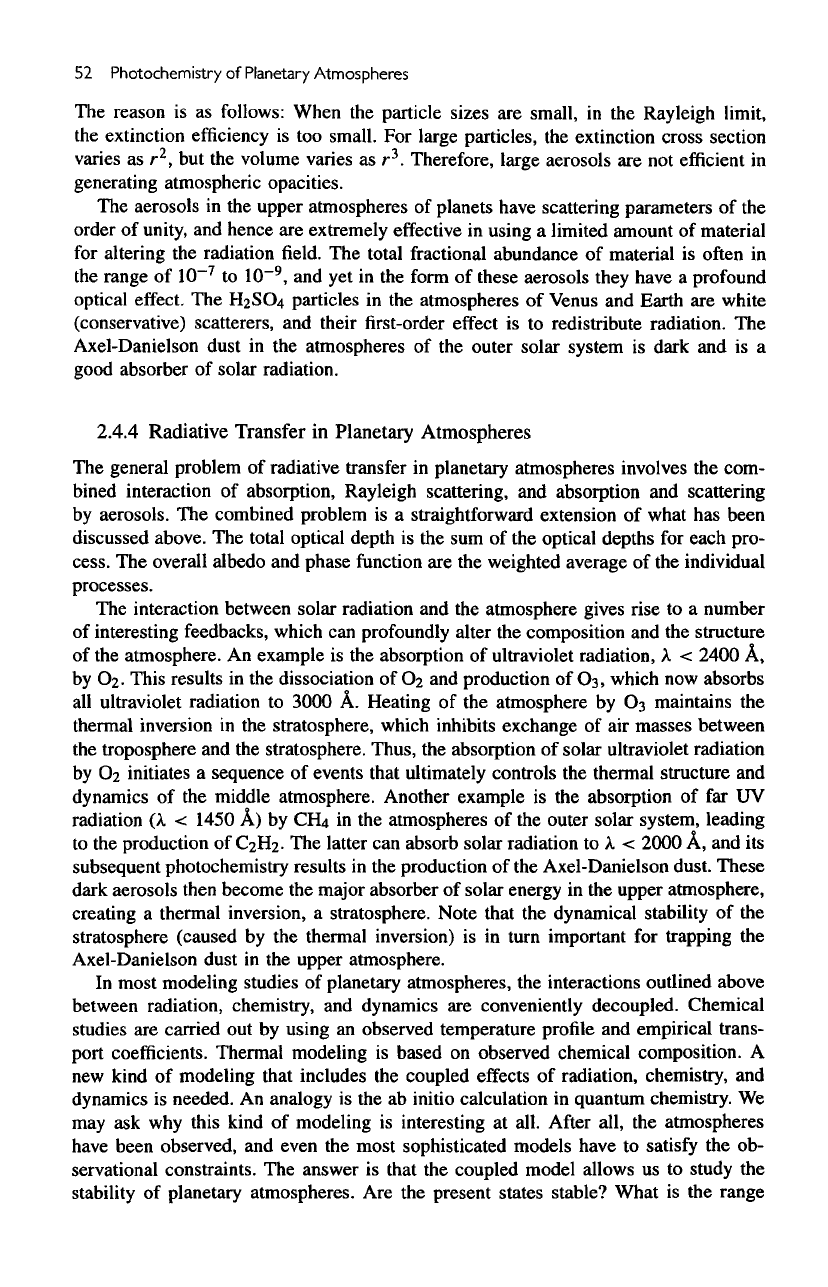
52
Photochemistry
of
Planetary Atmospheres
The
reason
is as
follows:
When
the
particle sizes
are
small,
in the
Rayleigh
limit,
the
extinction
efficiency
is too
small.
For
large particles,
the
extinction
cross
section
varies
as r
2
, but the
volume varies
as r
3
.
Therefore, large aerosols
are not
efficient
in
generating
atmospheric opacities.
The
aerosols
in the
upper atmospheres
of
planets have scattering parameters
of the
order
of
unity,
and
hence
are
extremely
effective
in
using
a
limited amount
of
material
for
altering
the
radiation
field. The
total fractional abundance
of
material
is
often
in
the
range
of
10~
7
to
10~
9
,
and yet in the
form
of
these
aerosols
they have
a
profound
optical
effect.
The
H2SO4 particles
in the
atmospheres
of
Venus
and
Earth
are
white
(conservative)
scatterers,
and
their
first-order
effect
is to
redistribute radiation.
The
Axel-Danielson
dust
in the
atmospheres
of the
outer solar system
is
dark
and is a
good absorber
of
solar radiation.
2.4.4
Radiative
Transfer
in
Planetary
Atmospheres
The
general problem
of
radiative transfer
in
planetary atmospheres involves
the
com-
bined
interaction
of
absorption, Rayleigh scattering,
and
absorption
and
scattering
by
aerosols.
The
combined problem
is a
straightforward extension
of
what
has
been
discussed above.
The
total optical depth
is the sum of the
optical depths
for
each pro-
cess.
The
overall albedo
and
phase
function
are the
weighted average
of the
individual
processes.
The
interaction between solar radiation
and the
atmosphere gives rise
to a
number
of
interesting feedbacks, which
can
profoundly alter
the
composition
and the
structure
of
the
atmosphere.
An
example
is the
absorption
of
ultraviolet radiation,
A.
<
2400
A,
by
O2.
This results
in the
dissociation
of
C>2
and
production
of
Os,
which
now
absorbs
all
ultraviolet radiation
to
3000
A.
Heating
of the
atmosphere
by 03
maintains
the
thermal inversion
in the
stratosphere, which inhibits exchange
of air
masses
between
the
troposphere
and the
stratosphere. Thus,
the
absorption
of
solar ultraviolet radiation
by
O2
initiates
a
sequence
of
events that ultimately controls
the
thermal structure
and
dynamics
of the
middle atmosphere. Another example
is the
absorption
of far
UV
radiation
(A.
<
1450
A) by CH4 in the
atmospheres
of the
outer solar system, leading
to the
production
of
C2H2-
The
latter
can
absorb solar radiation
to
A.
<
2000
A, and its
subsequent
photochemistry results
in the
production
of the
Axel-Danielson dust.
These
dark
aerosols
then
become
the
major absorber
of
solar energy
in the
upper atmosphere,
creating
a
thermal inversion,
a
stratosphere. Note that
the
dynamical stability
of the
stratosphere (caused
by the
thermal inversion)
is in
turn
important
for
trapping
the
Axel-Danielson
dust
in the
upper atmosphere.
In
most modeling studies
of
planetary atmospheres,
the
interactions outlined above
between
radiation, chemistry,
and
dynamics
are
conveniently decoupled. Chemical
studies
are
carried
out by
using
an
observed temperature profile
and
empirical trans-
port
coefficients.
Thermal modeling
is
based
on
observed
chemical composition.
A
new
kind
of
modeling that includes
the
coupled effects
of
radiation, chemistry,
and
dynamics
is
needed.
An
analogy
is the ab
initio
calculation
in
quantum chemistry.
We
may
ask why
this kind
of
modeling
is
interesting
at
all.
After
all,
the
atmospheres
have
been observed,
and
even
the
most sophisticated models have
to
satisfy
the ob-
servational constraints.
The
answer
is
that
the
coupled model allows
us to
study
the
stability
of
planetary atmospheres.
Are the
present
states
stable? What
is the
range