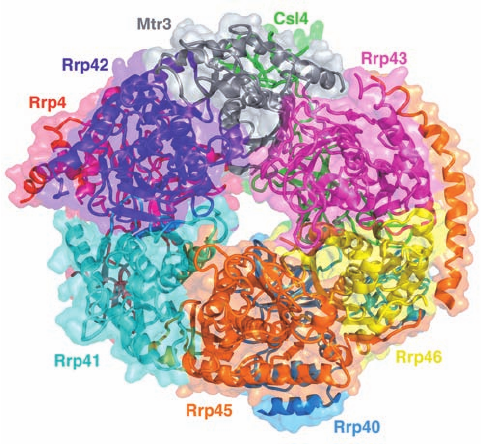
ated with mature mRNAs in the nucleus. However, it ap-
pears that it is its entire collection of bound proteins rather
than any individual protein that serves to identify an
mRNA to the nuclear export machinery.
The eukaryotic nucleus (Fig. 1-5) is a double membrane-
enveloped organelle that in animals is penetrated by an
average of ⬃3000 pores. These are formed by nuclear pore
complexes (NPCs), which are massive (⬃120,000 kD),
8-fold symmetric assemblies of ⬃30 different proteins
known as nucleoporins. NPCs, which have inner diameters
of ⬃90 Å (although this may be expandable to as much as
260 Å), allow the free diffusion of molecules of up to 50 kD,
but most macromolecules, including mRNAs in their com-
plexes with proteins, require an active transport process to
pass through an NPC. Some of the proteins associated with
mature mRNAs bear nuclear export signals that are recog-
nized by a protein receptor that in yeast is named Dbp5. This
482-residue DExD/H box protein (Dbp5 stands for
DExD/H box protein 5) is an ATP-driven RNA helicase that
also binds to the NPC.This permits Dbp5 to pull the mRNA
out into the cytosol while simultaneously stripping away
many of its bound proteins.These proteins are later recycled
by returning them to the nucleus through the NPCs.
v. mRNA Degradation Is Elaborately Controlled
The synthesis and maturation of mRNAs, as we have
seen, are subject to multiple controls. The same is true of
their degradation. Indeed, the range of mRNA stability in
eukaryotic cells, measured in half-lives, varies from a few
minutes to many hours or days. The mRNA molecules
themselves contain elements that dictate their decay rates.
These elements include the 3¿ poly(A) tail and the 5¿ m
7
G
cap, which protect against exonucleases, as well as se-
quences that are located within the coding region.
A major route for mRNA degradation begins with the
progressive removal of its poly(A) tail,a process catalyzed by
deadenylases that are located throughout the cytosol. When
the residual poly(A) tail is less than ⬃10 nt long and hence no
longer capable of binding poly(A) binding protein (Section
31-4Ab),the mRNA becomes a substrate for a decapping en-
zyme, which hydrolytically excises the mRNA’s m
7
G cap.
This is possible because the translational initiation factor
eIF4G interacts with both poly(A) binding protein and cap
binding protein (Section 32-3Cd), thereby circularizing the
mRNA so that events at its 3¿ end can be coupled to events at
its 5¿ end.The decapped and deadenylated mRNA is then de-
graded by exonucleases, mainly the 1706-residue 5¿S3¿ ex-
onuclease Xrn1 and the 3¿S5¿ exonuclease complex named
the exosome. A decapping enzyme,5¿S3¿ exonucleases, and
accessory proteins form P bodies (Section 31-4At) that func-
tion to either degrade mRNA or store it in an inactive form.
Proteins that bind to AU-rich elements (AREs) in the 3¿
untranslated region of mRNAs also appear to increase or
decrease the rate of mRNA degradation, although their ex-
act action is poorly understood. RNA secondary structure
and RNA-binding proteins, which may be susceptible to
modification by cellular signaling pathways, are thought to
play a role in regulating mRNA stability.
The eukaryotic core exosome consists of single copies of
nine different subunits. Its X-ray structure (Fig. 31-77), de-
termined by Christopher Lima, reveals that six of these
subunits, Rpr41 (Rpr for rRNA processing; the exosome
was discovered as an activity that processed the 3¿ ends of
rRNAs), Rrp42, Mtr3, Rrp43, Rrp46, and Rrp45, form a
six-membered ring with the remaining three subunits,
Rrp4, Csl4, and Rrp40, bound to the same face of this ring.
These subunits are arranged such that the core exosome
contains an ⬃9-Å-wide central channel that allows the en-
trance of only single-stranded RNAs.
The archeal exosome appears to be a simpler version of
the eukaryotic core exosome. Its six-membered ring consists
of only two types of subunits, Rrp41 and Rrp42, that alter-
nate around the ring, with three copies of Rrp4 bound to
the same face of the ring. Only Rrp41 contains an active
site although Rrp42 is required for activity. Not surpris-
ingly, eukaryotic Rrp4, Mtr3, and Rrp46 are homologs of
archeal Rrp41, eukaryotic Rrp42, Rrp43, and Rrp45 are
homologs of archeal Rrp42, and eukaryotic Rrp4, Csl4, and
Rrp40 are homologs of archeal Rrp4. Nevertheless, despite
the fact that each of its core subunits are essential for via-
bility, eukaryotic core exosomes, from yeast to humans, are
catalytically inactive due to changes in active site residues
relative to their archeal homologs. However, the core exo-
some associates with two 3¿-exonucleases, Rrp6 and Rrp44,
whose catalytically inactive mutants are individually viable
in yeast but lethal in combination. Moreover, the core exo-
some interacts with numerous mostly multisubunit cofac-
tors that carry out a variety of RNA processing activities in
both the nucleus and the cytosol.Thus, the eukaryotic core
exosome appears to be a structural platform upon which
many RNA processing enzymes can be mounted.
Section 31-4. Post-Transcriptional Processing 1327
Figure 31-77 X-ray structure of the human core exosome. The
protein complex is drawn in ribbon form embedded in its
semitransparent molecular surface, with each of its nine different
subunits separately colored.The view is toward the face of the
six-membered ring of subunits opposite that to which the three
other subunits bind. [Based on an X-ray structure by Christopher
Lima, Sloan-Kettering Institute, New York, New York. PDBid
2NN6.]
JWCL281_c31_1260-1337.qxd 8/11/10 9:49 PM Page 1327