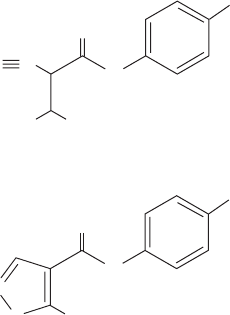
synthesize arginine via the urea cycle is synthesized by a
separate mitochondrial enzyme, carbamoyl phosphate syn-
thetase I (CPS I), which uses ammonia as its nitrogen
source. Prokaryotes only have one carbamoyl phosphate
synthetase, which supplies both pyrimidine and arginine
biosynthesis and utilizes glutamine. This latter enzyme, as
we have seen, contains three different active sites that are
connected by a remarkable 96-Å-long tunnel through
which intermediate products diffuse (Fig. 26-9).
The pyrimidine biosynthetic pathway is a target for
antiparasitic drugs. For example, the parasitic protozoan
Toxoplasma gondii, which infects most mammals, causes
toxoplasmosis, a disease whose complications include
blindness, neurological dysfunction, and death in immuno-
compromised individuals (e.g., those with AIDS). Most
parasites have evolved to take advantage of nutrients sup-
plied by their hosts. However, T. gondii is unable to meet its
needs exclusively through nucleotide salvage pathways and
retains the ability to synthesize uracil de novo. Drugs that
target the parasite’s carbamoyl phosphate synthetase II
(an enzyme whose structure and kinetics distinguish it
from its mammalian counterpart) could therefore prevent
T. gondii growth. Moreover, there is evidence that T. gondii
strains that have been engineered to lack carbamoyl phos-
phate synthetase II are avirulent and could be useful as
vaccines in humans and livestock.
2. Synthesis of carbamoyl aspartate. Condensation of
carbamoyl phosphate with aspartate to form carbamoyl
aspartate is catalyzed by aspartate transcarbamylase
(ATCase). This reaction, the pathway’s flux-generating
step, occurs without need of ATP because carbamoyl phos-
phate is intrinsically activated. The structure and regula-
tion of E. coli ATCase is discussed in Section 13-4.
3. Ring closure to form dihydroorotate. The third reac-
tion of the pathway was elucidated by Arthur Kornberg
following his observation that microorganisms made to uti-
lize orotic acid as a carbon source first reduce it to dihy-
droorotate. The reaction forming the pyrimidine ring
yields dihydroorotate in an intramolecular condensation
catalyzed by the zinc metalloenzyme dihydroorotase.
4. Oxidation of dihydroorotate. Dihydroorotate is irre-
versibly oxidized to orotate by dihydroorotate dehydroge-
nase (DHODH). The eukaryotic enzyme, which contains
FMN, is an integral membrane protein that is located on
the outer surface of the inner mitochondrial membrane,
where ubiquinone supplies its oxidizing power. The other
five enzymes of pyrimidine nucleotide biosynthesis are
cytosolic in animal cells. Many bacterial dihydroorotate de-
hydrogenases are NAD
-linked flavoproteins that contain
FMN, FAD, and a [2Fe–2S] cluster. These enzymes nor-
mally function degradatively, that is, in the direction oro-
tate S dihydroorotate, thereby permitting these bacteria
to metabolize orotate and accounting for Kornberg’s ob-
servation. The reaction mediated by eukaryotic DHODH
involves two redox steps, as is indicated in Fig. 28-8. The
X-ray structure of human DHODH in complex with oro-
tate, determined by Jon Clardy, reveals that the pyrimidine
ring of orotate is stacked over the FMN’s flavin ring with
the orotate C6 and FMN N5 separated by 3.6 Å, a distance
that is compatible with direct hydride transfer between
these two centers. A tunnel leads from the opposite side of
the flavin ring to a hydrophobic region on the enzyme sur-
face. The enzyme presumably binds to the mitochondrial
membrane surface via this hydrophobic patch, thereby per-
mitting ubiquinone, which readily diffuses within the mito-
chondrial membrane, to approach and reoxidize the en-
zyme’s bound FMNH
2
. In the X-ray structure, this tunnel
contains a tightly bound molecule named A77 1726, which
is the primary metabolite of leflunomide (trade name
Arava),
a compound that is in clinical use for the treatment of
rheumatoid arthritis. A77 1726 attenuates this autoimmune
disease by blocking pyrimidine biosynthesis in T lympho-
cytes, thereby reducing their inappropriate proliferation.
However,A77 1726 does not inhibit bacterial DHODHs.
5. Acquisition of the ribose phosphate moiety. Orotate
reacts with PRPP to yield orotidine-5-monophosphate
(OMP) in a reaction catalyzed by orotate phosphoribosyl-
transferase and driven by hydrolysis of the eliminated PP
i
.
This reaction fixes the anomeric form of pyrimidine nu-
cleotides in the configuration. Orotate phosphoribosyl-
transferase also acts to salvage other pyrimidine bases,
such as uracil and cytosine, by converting them to their cor-
responding nucleotides. Although the various phosphori-
bosyltransferases, including HGPRT, exhibit little se-
quence similarity, their X-ray structures indicate that they
contain a common structural core that resembles the dinu-
cleotide binding fold (Section 8-3Bi) but lacks one of its
strands.
6. Decarboxylation to form UMP. The final reaction of
the pathway is the decarboxylation of OMP by OMP de-
carboxylase (ODCase) to form UMP. ODCase enhances
the rate (k
cat
/K
M
) of OMP decarboxylation by a factor of
2 10
23
over that of the uncatalyzed reaction, making it the
most catalytically proficient enzyme known. Yet ODCase
has no cofactors to help stabilize the reaction’s putative
carbanion intermediate. How is it able to do so? The X-ray
structure, by Steven Ealick, of ODCase from B. subtilis in
complex with UMP indicates that a bound OMP’s C6
A77 1726
O
O
CN
N
O
CH
3
CH
3
CF
3
HO
N
H
N
H
CF
3
Leflunomide
1116 Chapter 28. Nucleotide Metabolism
JWCL281_c28_1107-1142.qxd 4/22/10 9:16 AM Page 1116