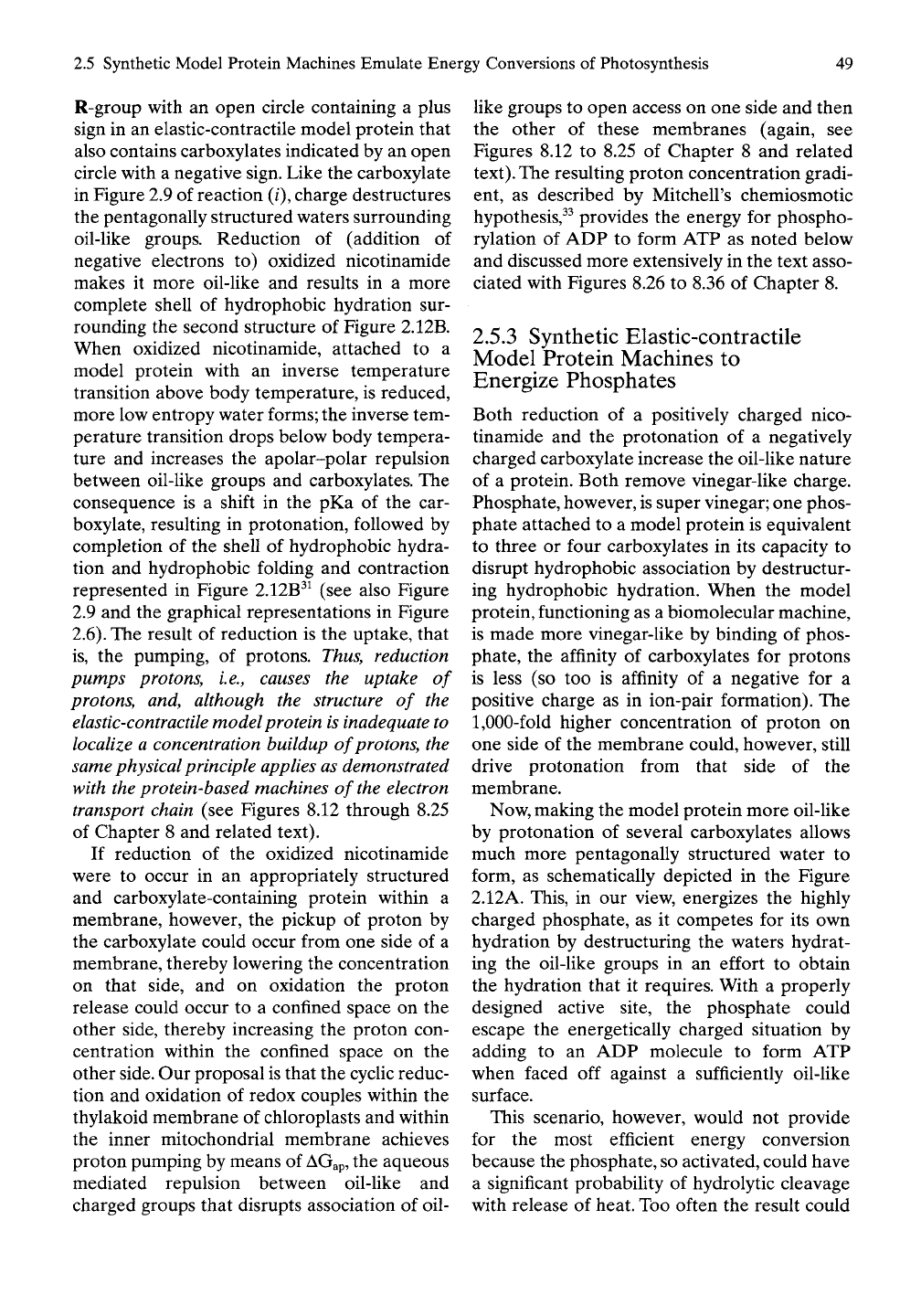
2.5 Synthetic Model Protein Machines Emulate Energy Conversions of Photosynthesis
49
R-group with an open circle containing a plus
sign in an elastic-contractile model protein that
also contains carboxylates indicated by an open
circle with a negative sign. Like the carboxylate
in Figure 2.9 of reaction (/), charge destructures
the pentagonally structured waters surrounding
oil-like groups. Reduction of (addition of
negative electrons to) oxidized nicotinamide
makes it more oil-like and results in a more
complete shell of hydrophobic hydration sur-
rounding the second structure of Figure 2.12B.
When oxidized nicotinamide, attached to a
model protein with an inverse temperature
transition above body temperature, is reduced,
more low entropy water forms; the inverse tem-
perature transition drops below body tempera-
ture and increases the apolar-polar repulsion
between oil-like groups and carboxylates. The
consequence is a shift in the pKa of the car-
boxylate, resulting in protonation, followed by
completion of the shell of hydrophobic hydra-
tion and hydrophobic folding and contraction
represented in Figure 2.12B^^ (see also Figure
2.9 and the graphical representations in Figure
2.6).
The result of reduction is the uptake, that
is,
the pumping, of protons. Thus, reduction
pumps protons, i.e., causes the uptake of
protons, and, ahhough the structure of the
elastic-contractile model protein is inadequate to
localize a concentration buildup of protons, the
same physical principle applies as demonstrated
with the protein-based machines of the electron
transport chain (see Figures 8.12 through 8.25
of Chapter 8 and related text).
If reduction of the oxidized nicotinamide
were to occur in an appropriately structured
and carboxylate-containing protein within a
membrane, however, the pickup of proton by
the carboxylate could occur from one side of a
membrane, thereby lowering the concentration
on that side, and on oxidation the proton
release could occur to a confined space on the
other side, thereby increasing the proton con-
centration within the confined space on the
other side. Our proposal is that the cyclic reduc-
tion and oxidation of redox couples within the
thylakoid membrane of chloroplasts and within
the inner mitochondrial membrane achieves
proton pumping by means of
AGap,
the aqueous
mediated repulsion between oil-like and
charged groups that disrupts association of oil-
like groups to open access on one side and then
the other of these membranes (again, see
Figures 8.12 to 8.25 of Chapter 8 and related
text).
The resulting proton concentration gradi-
ent, as described by Mitchell's chemiosmotic
hypothesis,^^ provides the energy for phospho-
rylation of ADP to form ATP as noted below
and discussed more extensively in the text asso-
ciated with Figures 8.26 to 8.36 of Chapter 8.
2.5.3 Synthetic Elastic-contractile
Model Protein Machines to
Energize Phosphates
Both reduction of a positively charged nico-
tinamide and the protonation of a negatively
charged carboxylate increase the oil-Hke nature
of a protein. Both remove vinegar-like charge.
Phosphate, however, is super vinegar; one phos-
phate attached to a model protein is equivalent
to three or four carboxylates in its capacity to
disrupt hydrophobic association by destructur-
ing hydrophobic hydration. When the model
protein, functioning as a biomolecular machine,
is made more vinegar-like by binding of phos-
phate, the affinity of carboxylates for protons
is less (so too is affinity of a negative for a
positive charge as in ion-pair formation). The
1,000-fold
higher concentration of proton on
one side of the membrane could, however, still
drive protonation from that side of the
membrane.
Now, making the model protein more oil-hke
by protonation of several carboxylates allows
much more pentagonally structured water to
form, as schematically depicted in the Figure
2.12A. This, in our view, energizes the highly
charged phosphate, as it competes for its own
hydration by destructuring the waters hydrat-
ing the oil-like groups in an effort to obtain
the hydration that it requires. With a properly
designed active site, the phosphate could
escape the energetically charged situation by
adding to an ADP molecule to form ATP
when faced off against a sufficiently oil-like
surface.
This scenario, however, would not provide
for the most efficient energy conversion
because the phosphate, so activated, could have
a significant probability of hydrolytic cleavage
with release of heat. Too often the result could